Immunization to
Protect the U.S. Armed Forces:
Heritage, Current
Practice, Prospects
John D. Grabenstein,
RPh, PhD *
Phillip R. Pittman,
MD, MPH †
John T. Greenwood,
PhD *
Renata J. M. Engler,
MD #
* Office of the
Surgeon General, U. S. Army, Falls
Church, Virginia
† U.S. Army Medical
Research Institute of Infectious
Diseases, Fort Detrick, Maryland
# Allergy-Immunology
Department, Vaccine Healthcare
Centers Network, Walter Reed
Army Medical Center,
Washington, DC
The opinions and
assertions contained herein are
those of the authors and are not to
be construed as official or as
reflecting the views of the U.S.
Army or the Department of Defense.
Immunization to
Protect the U.S. Armed Forces:
Heritage, Current Practice,
Prospects
Immunization
protects the personal health of
United States military personnel and
maintains their mission readiness.
The immunization program of the U.S.
Department of Defense (DoD) is
broad-ranging, protecting the forces
from an array of pathogenic threats.
Because the active and reserve
components of the U.S. DoD consist
of over 2.2 million people at any
given time, the program immunizes a
significant percentage of the U.S.
adult population. This article
updates and expounds on previous
reviews of the U.S. military
immunization program, (1-7)
discussing historical perspectives,
the rationale for current
immunization policies, and future
prospects. Military immunization
requirements often exceed those
provided to civilian adults, because
of the travel and other occupational
hazards confronted by soldiers,
marines, sailors, airmen, and coast
guardsmen. Military immunization
requirements are quite similar for
each of the five Armed Forces (i.e.,
Army, Marine Corps, Navy, Air Force,
Coast Guard). The requirements and
recommendations are described in a
joint immunization regulation, (8)
summarized in table 1.
Immunizations have
both direct benefit to the recipient
and indirect benefit to the people
in the community the vaccinee
resides in or works with (i.e.,
“herd immunity”). “Herd immunity” or
“community immunity” results when a
decreased number of susceptible
people and the decreased excretion
of infectious particles impairs
disease transmission. In military
settings, the indirect benefit takes
on an additional dimension, insofar
as an immunized service member is
less likely to succumb to a disease
that threatens his or her team’s
mission. By staying healthy, the
immunized service member helps other
team members accomplish their
mission and return home safely. Due
to both direct and indirect
benefits, most U.S. military
immunizations are required, rather
than voluntary. Figures 1 and 2
illustrate records used to document
immunizations of troops during World
War II.
Senior
preventive-medicine officers from
the five Armed Services develop
vaccine recommendations for military
trainees and other military
personnel, with decisions made by
the Army, Navy, and Air Force
Surgeons General and the Coast Guard
Director of Health & Safety.
Immunization policies consider
epidemic potential, exposure risk
(e.g., travel, occupation), and the
potential for contingency work in
unsanitary conditions. During policy
development, advice may be sought
from the Armed Forces
Epidemiological Board (AFEB), an
expert advisory board of civilian
physicians and scientists. (9, 10)
Before the Food & Drug
Administration (FDA) took its
present dominant role in vaccine
regulation in the mid-1970s, the
AFEB’s cutting-edge expertise was
pivotal in deciding immunization
dosing schedules and vaccine
composition.
Modern immunization
policy development takes into
account public-health
recommendations published by the
Centers for Disease Control and
Prevention (CDC), in consultation
with its Advisory Committee on
Immunization Practices (ACIP).
Recommendations, guidelines, and
disease-surveillance information are
also considered from other agencies
and expert bodies, such as the
National Vaccine Advisory Committee,
the American Academy of Pediatrics,
the American College of Physicians,
the American College of
Obstetricians and Gynecologists, the
World Health Organization, and
others. With respect to malicious
infections and bioweapons,
risk-assessment information is
gathered from the Defense
Intelligence Agency, the Armed
Forces Medical Intelligence Center,
the Department of Homeland Security
and similar organizations.
A synopsis of
vaccines commonly administered at
various historical points to U.S.
military personnel appears in table
2. (1-18) The U.S. military
contributions to vaccine development
are summarized in table 3.
The following
sections, grouped categorically,
review major vaccine-preventable
infectious threats to military
personnel. These reviews begin with
smallpox, a disease that spans
several of these categories during
the last 230 years.
SMALLPOX The first
immunization program implemented for
the U.S. military was variolation
(i.e., variola inoculation) of
trainees entering the Continental
Army in 1777. (3, 11, 13, 16, 19-23)
Impetus for this program began in
fall 1775, as British forces
expelled smallpox cases and recently
variolated people from Boston, and
sent them across siege lines
maintained by the fledgling
Continental Army. In the winter of
1775-76, up to half of the
Continental Army task force
advancing on Québec was ill with
smallpox. The Americans suffered
5,500 smallpox casualties among
their force of 10,000 colonial
troops. Major General John Thomas,
their commander, died of smallpox
during the campaign, as did many
other soldiers. Decimated, the U.S.
forces lost the Battle of Québec and
were obliged to retreat in May 1776.
Arguably, the British colony of
Canada was not incorporated into the
fledgling United States because of
this smallpox outbreak.
In response to the
military defeat outside Québec, John
Morgan, the Director General of the
Army Hospital, along with his
successor William Shippen, Jr., and
Benjamin Rush (influential physician
and signer of the Declaration of
Independence), recommended to George
Washington that the Continental Army
be variolated. (16, 23) Variolation
was an archaic and dangerous method
of preventing smallpox with a 1 to
2% mortality risk, but the best
method then available. Variolation
involved applying smallpox-infected
material, such as ground-up scabs,
to an incision of the skin to induce
immunity. Viral particles embedded
in fibrin scab were felt to be less
virulent than direct exposure to
lesions or droplets.
Variolation had its
roots in Africa and Asia. First
introduced in England in 1719, the
mortality from intentional
variolation could reach as high as
12%, particularly if people were
pretreated with bleeding and
fasting. Further, variolated people
could spread smallpox to others for
several weeks after the procedure.
Washington was reluctant to initiate
such a drastic, unpredictable
measure. Variolation was attended by
rumors of serious complications.
Respected leaders like Benjamin
Franklin opposed the practice;
Franklin later changed his view
after his son died of smallpox. (19,
23)
At Morristown, New
Jersey, in January 1777, Washington
finally ordered a mandatory
inoculation program for his troops
(principally new recruits), if they
had not survived smallpox infection
earlier in life, because the lethal
risk from infection (~16%) was
judged far greater than from
variolation (1 in 300). (16) “Should
the disorder infect the Army in a
natural way and rage with its usual
virulence, we should have more to
dread from it than from the sword of
the enemy.” (22)
America’s
Continental Army was thus the first
army in the world to adopt an
organized program requiring smallpox
inoculation, with measurable
reductions in both morbidity rates
and mortality (to less than 1%).
Within the British Army, variolation
was voluntary. To prevent smallpox
the disease from spreading via
secondary contact with variolated
troops, Washington and his medical
leaders performed the procedure in
“inoculation hospitals” and isolated
the troops in vaccination huts. (11,
19, 23) For examples, recruits
passing through Virginia to join the
Army were inoculated at Alexandria.
General Washington’s variolation
policy enabled a fighting force
strong enough to achieve the
surrender of British units at
Saratoga. Had variolation been
adopted earlier, an American victory
at Québec could have hastened the
end of the Revolution. (3, 11, 16)
Variolation was
later replaced by vaccination with
cowpox virus. Edward Jenner’s 1798
report that intentionally injecting
the less virulent cowpox (now
vaccinia) virus would cross-protect
against variola virus represented a
major advance in vaccine safety. (4,
13, 19) Jenner’s success met
stubborn resistance among some,
related to safety and ethical
concerns. But the value of
vaccination was readily apparent and
soon made its way across the
Atlantic Ocean. For the War of 1812,
the U.S. War Department ordered that
vaccination be substituted for
variolation to prevent smallpox.
(11, 16) In 1848, the U.S. Navy did
the same.
Smallpox vaccination
was gradually, but incompletely,
adopted among the civilian populace.
But smallpox remained an endemic and
sometimes epidemic disease in the
United States and around the globe.
During the American Civil War, use
of smallpox vaccine expanded on both
sides of the conflict, including in
training camps. Nonetheless, an
estimated 19,000 cases of smallpox
occurred among the troops, with ~
7,000 deaths. (4, 12, 16, 24, 25)
In France in 1869,
an estimated 200,000 people died of
smallpox. (19) During the Franco-
Prussian War of 1870-71, the
Prussian Army of 800,000 men
revaccinated their personnel every 7
years. The Prussians suffered 8,463
cases of smallpox, with a
case-fatality ratio of 5.4%. In
contrast, the French Army was
unvaccinated. They suffered 125,000
cases of smallpox, with a fatality
rate if infected of 18.7%. In the
Spanish-American War of 1898,
volunteer troops were vaccinated
against smallpox as they mustered
into service. More smallpox cases
occurred among these volunteers than
among the Regular Army. Most of the
825 smallpox admissions (258 fatal)
occurred in the Philippine Islands
during that conflict. (4, 12, 16,
19, 25)
Military training
camps continued to administer
smallpox vaccine during World War I.
(26) There were 853 smallpox
admissions (780 within the United
States) with 14 deaths. In 1919, an
American citizen named Charles
Higgins sent an angry and lengthy
manuscript to President Woodrow
Wilson, pleading that he stop
smallpox vaccination for Armed
Forces, listing both true and
erroneous risks of smallpox
vaccination. (27) But vaccination
continued through the decades,
successfully, leading eventually to
a global vaccination program that
eradicated naturally occurring
smallpox infection from the planet.
(19)
The United States
military conducted major smallpox
vaccination programs during World
War II, for its own personnel and
for local populations at risk of
smallpox. (14, 15, 26) Among U.S.
troops, there were 115 smallpox
cases (105 overseas). Cases were
attributed to three main causes:
Failure to vaccinate properly, an
inadequate cold chain to keep the
vaccine potent, and failure to read
and interpret vaccination reactions
properly. Additional cases occurred
shortly after the war, accompanying
large population movements returning
to the Japanese home islands or
returning to the Korean peninsula.
Among the last Americans to contract
smallpox were five Soldiers, one of
whom died, during the 1953 smallpox
outbreak amid the Korean War. By the
early 1970’s, with smallpox not
circulating within the United
States, routine smallpox vaccination
of civilians (especially children)
was no longer practiced, because
complications like eczema vaccinatum
and encephalitis were not considered
justified risks in the face of no
disease threat. (19) US service
members were routinely vaccinated
against smallpox until 1984. (1, 28)
In 1984, routine
military smallpox vaccinations were
limited to new troops entering basic
training. Between 1984 and 1990,
smallpox vaccinations at basic
training sites were intermittent,
due to a shortage of vaccinia immune
globulin, to treat certain adverse
events after vaccination, pressure
from civilian authorities (who
perceived the variola threat as a
weapon to be negligible), and the
appearance of the novel clinical
entity of HTLV-III infection (now
called human immunodeficiency virus,
HIV). (9) In March 1990, the
Department of Defense “temporarily
discontinued” smallpox vaccination
of basic trainees, even though
trainees were essentially in medical
quarantine. (29) Military
vaccination was limited to special
circumstances, such as for
laboratory workers exposed to other
orthopox viruses. This “temporary”
policy seemed to be a permanent
state of affairs, until the anthrax
attacks along the eastern seaboard
in fall 2001 heightened concerns
about bioterrorism generally.
President Bush announced a national
smallpox vaccination program on
December 13, 2002. (28)
In this plan,
smallpox vaccinations resumed for
medical and epidemic response teams,
and for troops deployed to
high-threat areas. Troops were
included to protect them in case of
a bioweapon attack and enable them
to continue their missions. Two
hundred years after Edward Jenner
popularized the use of an orthopox
virus to cross protect against
variola, vaccinia vaccine was called
into service again. The current
program is described below, in the
bioweapon countermeasure section.
PROPHYLAXIS OF
DISEASES RELATED TO POOR HYGIENE OR
SANITATION
During military
deployments to developing countries
or military exercises, the risk of
contracting endemic infections is a
serious concern. Some of these
infections may not be evident until
the deployment is well underway or
even after return to the United
States, for infections with
prolonged incubation periods. During
actual conflict, sanitation is often
compromised and wound and
blood-borne infections become a
greater hazard to military forces.
The science of vaccinology advanced
slowly, so smallpox vaccine was the
only vaccine available for nearly
the whole century. A few troops may
have received post-exposure
prophylaxis against rabies using
Pasteur’s vaccine. (30) Endemic
diseases that today can be prevented
by immunization took a heavy toll on
military rosters, despite aggressive
efforts at promoting personal
hygiene and maintaining proper
sanitation in the camps. Measles,
hepatitis, typhoid, dysentery,
malaria, and typhus were significant
causes of non-battle-related
illnesses. But by the 1890s, an
awareness of the potential benefits
of immunization was increasing.
Beginning as early
as the Spanish-American War or 1898,
the U.S. Army established scientific
advisory boards to address issues
such as typhoid, yellow fever,
malaria, dengue fever, pneumonia,
and influenza. (2, 16, 25) These
boards were crucial in the
development of specific preventive
strategies and research efforts in
vaccine development. Preventive
medicine gained new prominence as a
contributor to the war effort during
World War I, but the lack of
antibiotics or specific vaccines
forced a reliance on passive
immunization via antitoxins.
Diphtheria antitoxin was an
important form of therapy. (4)
Antitoxins to treat gas gangrene
(i.e., Clostridium perfringens or
welchii and related organisms),
botulism, and dysentery (i.e.,
Shigella dysenteriae or flexneri)
provided some therapeutic value. (4,
7, 15) Antibiotics largely replaced
these products by the end of World
War II.
Typhoid fever
In 1898, the
battleship USS Maine blew up in
Havana harbor, leading to the
Spanish- American War. Volunteer
soldiers, their officers, and
physicians marched to the trainee
camps, where they began to get sick
and die. Army Surgeon General George
M. Sternberg appointed Major Walter
Reed to lead an investigative team.
Building on John Snow’s observations
on cholera in London, the team
showed the cause to be typhoid
fever, due to contamination of the
water supply. During the war,
America experienced 280 battle
fatalities. But at the five main
training camps, before getting
anywhere near a battlefield, there
were 20,738 cases of typhoid fever,
1,590 of them fatal. In the Army
overall, there were an estimated
2,620 typhoid fever deaths. (1, 2,
3, 16, 25, 31-35)
During the late
1800s and early 1900s, scientists
developed vaccines against
infections such as typhoid fever,
cholera, plague, and rabies. Almroth
Wright developed a typhoid vaccine
in Britain, based on the hypothesis
that dead bacilli could evoke a
protective antibody response. (36,
37) The British Army used early
forms of typhoid vaccine during the
Anglo-Boer War in southern Africa in
1899. Among 14,626 immunized British
soldiers, there were 1,417 cases of
typhoid fever and 163 deaths
(11/1,000 soldiers). In contrast,
among 313,618 unimmunized soldiers,
there were 48,754 cases and 6,991
deaths (32/1,000). (38) Of the
cases, 19,454 were invalided back to
the British Isles for discharge with
a medical pension. Lieutenant
Colonel William Leishman continued
this research in the United Kingdom,
standardizing production methods and
performing studies to show by 1908
that two typhoid immunizations gave
excellent protection.
Major Frederick
Russell of the Army Medical School
adapted British and German
production methods to produce the
inactivated whole-cell typhoid
vaccine supply for the entire United
States. The Army Medical School was
the first school of preventive
medicine and public health in the
United States and is the forerunner
of today’s Walter Reed Army
Institute of Research (WRAIR) and
Uniformed Services University of
Health Sciences. Voluntary
immunization of some American
soldiers against typhoid fever began
in 1909. (4, 12, 13, 16, 39-41) In
1911, Army Chief of Staff Major
General Leonard Wood (who began his
Army career as a military physician)
ordered mandatory typhoid
immunization for a 15,000-man
division, using Russell’s vaccine.
Wood also required that
immunizations be recorded in medical
records. Only two cases of typhoid
fever occurred. A few months later,
immunization became mandatory for
all soldiers. The Navy adopted
typhoid immunization as well.
With a vaccine to
shield troops from typhoid bacteria
during World War I, a mere ~ 2,000
cases of typhoid fever, with 227
deaths, were reported among 4.1
million Americans in uniform. (4,
12, 13, 42) The tragically high
rates of typhoid morbidity and
mortality during the
Spanish-American War faded to nearly
nothing by World War I. Had the
typhoid incidence rates of 1898 been
applied to the troops mobilized for
World War I, there would have been
500,000 cases and over 50,000
deaths. (15, 32, 43) Various vaccine
combinations of typhoid, paratyphoid
A, and paratyphoid B antigens were
used during the 20th century. (4,
12, 42, 43) The trivalent vaccine
was commonly known as TAB vaccine,
triple vaccine, or enteric vaccine.
Inactivated by heat and phenol, the
vaccine contained whole-cell
preparations of
Salmonella typhi, Salmonella
paratyphi
(A),
and
Salmonella schottmuelleri
(B).
In 1916, the U.S. Army Medical
School discovered paratyphoid A and
B bacilli and developed a vaccine
against them. But the paratyphoid
components were of questionable
efficacy. In the following decades,
TAB vaccine was withdrawn,
reintroduced, and then withdrawn
again. In 1940, the triple TAB
formulation was relicensed. Around
1945, paratyphoid A & B components
were removed again from U.S.
formulations. Typhoid fever affected
0.42 cases per thousand soldiers in
World War I, but only 0.05 cases per
thousand in World War II, due to
expanded immunization.
Various typhoid
vaccines were developed at WRAIR,
notably the acetone-killed and dried
(AKD) and heat-phenol (H-P) treated
formulations. (3, 7, 44) The AFEB
preferred the AKD vaccine for its
clinical efficacy. (9) Today, clean,
chlorinated water supplies (a
technique developed in 1910 by Army
Major (later BG) Carl R. Darnall)
(16) and other public-health
practices prevent typhoid bacteria
from entering military training
camps (and cities) in the United
States. The risk for typhoid fever
is encountered overseas, so that
typhoid immunization is standard
before overseas deployments, but not
part of trainee immunization itself.
Development of the typhoid Vi
polysaccharide injectable vaccine
and live attenuated typhoid vaccine
capsules rendered obsolete the
whole-cell typhoid vaccines,
renowned for high degrees of
injection-site pain and swelling,
fever, and systemic reactions. (7,
45)
Tetanus
Traumatic injuries
complicated by tetanus spores within
wounds were a major cause of
morbidity and mortality until World
War I. Passive immunization with
tetanus antitoxin was relatively
effective. But it had a harsh
side-effect profile, notably a
systemic hypersensitivity reaction
known as serum sickness, due to its
equine protein content. In 1933,
tetanus toxoid was licensed in the
United States, but was adopted
slowly for the civilian populace.
Tetanus toxoid offered the ability
to prevent tetanus, rather than
treating it after a wound occurred.
On the other hand, many more people
needed to receive a prophylactic
intervention, compared to a
therapeutic one. Using active rather
than passive immunization for
tetanus was another example of
immunization as a means of planning
ahead to prevent disease. (4, 7, 12,
13, 43, 46)
The Army Surgeon
General requested use of tetanus
toxoid for all active-duty American
troops in May 1940. (3, 4, 14, 15,
47, 48) Routine tetanus immunization
was approved by the War Department
in June 1941. This decision featured
the adoption of a promising new
technology that had not been widely
used. A record of tetanus toxoid
doses administered was stamped on
Soldiers' identification tags (e.g,
“T 41-42”), as well as in paper
records. (14) The example in Figure
3 reflects tetanus immunizations in
1942 and 1944. Booster toxoid doses
were routinely given before entering
an overseas theater and following a
wound. Many duplicative
immunizations resulted when records
were not forwarded with the troops
upon deployment to a new theater.
The incidence of local reactions
after immunization increased when
booster doses were administered at
short intervals. The U.S. Navy
tended to use alum-precipitated
toxoid, which induced more
persistent antibody concentrations,
compared to the fluid toxoid used by
the U.S. Army at that time. (49)
Only 12 cases of
tetanus were reported throughout
World War II, from all theaters of
operations, despite more than 12
million Americans in uniform who
incurred more than 2.7 million
hospital admissions for wounds or
injuries. (3, 4, 47) All 12 cases
were in unimmunized or incompletely
immunized troops. Across the
Atlantic, the German Army (the
Wehrmacht) did not give tetanus
toxoid to its troops, continuing to
rely on now-obsolete tetanus
antitoxin. (4) The Wehrmacht
suffered high rates of morbidity and
mortality from tetanus. In contrast,
the German Air Force (the Luftwaffe)
immunized its men with tetanus
toxoid and suffered much less
morbidity and mortality.
Today,
tetanus-diphtheria (Td) toxoids are
administered to all trainees upon
entry into military service. The
recently licensed
tetanus-diphtheria-acellular
pertussis (Tdap) vaccines will
likely warrant switching to Tdap, to
prevent prolonged cough illness
among service members, and provide
indirect benefit to service members’
children. (50, 51) Td (or soon Tdap)
booster immunizations are given
every 10 years to military members,
consistent with ACIP
recommendations. People who may not
have received a basic immunizing
series earlier in life receive a
complete series.
Hepatitis A
During the second
year of the Civil War, an estimated
5% of Union troops were jaundiced.
During World War II, 180,000 troops
developed infectious hepatitis
(i.e., hepatitis A), principally in
North Africa, Italy, the South
Pacific, and post-war Germany.
Within the Pusan perimeter during
the Korean War, 4,000 Americans were
hospitalized with hepatitis. (4, 14,
17, 52, 53) Edwin Cohn, John Oncley,
and colleagues at Harvard University
isolated the gammaglobulin fraction
of serum in 1944, under contract to
the U.S. Navy. Their methods 6
and 9, featuring a cold-ethanol
fractionation process, yielded
immune globulin for intramuscular
administration (IGIM, “gamma
globulin”). Joseph Stokes, Jr., and
John Neefe reported the utility of
IGIM in reducing the incidence of
hepatitis A in 1945. Clinicians
primarily used this drug to prevent
or mitigate measles, mumps,
hepatitis A, and hepatitis B. (1, 2,
3, 6, 7, 9, 10, 46, 52-57) IGIM
prevented post-transfusion hepatitis
in battle casualties, if given
promptly. (58) IGIM was routinely
given to troops assigned to Korea or
Vietnam in the 1960s. (9, 57, 59-62)
In 1985, COL Leonard
Binn and colleagues tested a
formalin-inactivated hepatitis A
vaccine at WRAIR. They began a
collaborative research program with
SmithKline Beecham (SKB). Merck
Vaccine Division also built on the
work of WRAIR scientists in
developing its hepatitis A vaccine.
In 1991, LTC Bruce Innis and a team
of WRAIR scientists began an
efficacy trial of the SKB vaccine in
Thai school children. In 1991,
Werzberger and colleagues began an
efficacy trial of Merck’s vaccine in
upstate New York. (7, 53, 63, 64)
Hepatitis A
prophylaxis was transformed in
February 1995, with the U.S.
licensing of SKB’s inactivated
hepatitis A vaccines, rendering
obsolete serial painful injections
of IGIM into the buttocks. The FDA
licensed Merck’s vaccine in 1996.
With hepatitis A infection the most
common vaccine-preventable infection
among international travelers, (7,
10, 65, 66) a universal
immunization policy for U.S.
military personnel was a logical
step.
Hepatitis B
In 1942, infectious
hepatitis and serum hepatitis were
first clearly differentiated. This
came about when some lots of
yellow-fever vaccine were
unknowingly manufactured with
tainted serum albumin, added as a
stabilizer (discussed below). We now
know these two forms of liver
disease to be caused by hepatitis A
and hepatitis B viruses,
respectively. (9, 52, 53, 67-69) No
immunologic means of preventing
hepatitis B were available until the
FDA licensed the first hepatitis B
vaccine in 1981. IGIM was found to
provide temporary prophylaxis
against both hepatitis A and
hepatitis B infections in troops
assigned to Korea. (53, 57, 61)
Today, hepatitis B immunization
policy for U.S. military personnel
focuses on several cohorts at
occupational risk (e.g., medical and
mortuary affairs). Personnel
assigned to the Korea peninsula or
other high-risk areas since 1986
have been immunized, as have
accessions since 2001. (9, 53, 70)
Because of the vaccine’s relatively
high cost, hepatitis B vaccine
initially was administered
intradermally, but this route of
administration fell out of favor
nationally with several vaccines
(e.g., hepatitis B, rabies), due in
part to inconsistent injection
technique and antibody response. (7,
71, 72)
Cholera
The first injectable
cholera vaccines date back to the
1890s. The bacterial strains used in
the U.S. were selected at the Army
Medical School during World War II.
(3) From the 1940s to the 1980s,
injectable whole-cell cholera
vaccine was given to alert units.
But the vaccine fell into disfavor
as cholera immunization ceased being
a condition for passage of
international borders, as well as
the vaccine’s tendency to evoke
substantial injection-site and
systemic reactions, modest efficacy
(50% disease reduction), and short
duration of protection (~ 6 months).
The AFEB recommended cessation of
routine cholera immunization in
1973. (9) Emphasis on sanitation is
now considered more important in a
healthy adult military population
than reliance on a vaccine with
limited efficacy. Still, if supply
or transport disruptions stranded an
American military force in a
cholera-endemic area, widespread
disease outbreaks could occur. At
present, the oral cholera vaccines
developed under US Army contract by
the University of Maryland and
licensed in Europe and Canada are
unlicensed in the United States. (7,
33, 73, 74)
PROPHYLAXIS OF
CONTAGION & EPIDEMICS
Until 1990, loss of
life due to non-traumatic causes had
decimated more armies than bullets
in American military campaigns. (16,
75) The Russo-Japanese war of
1904-05 was a notable exception.
Military personnel encounter disease
threats in several settings: during
training, deployments, mobilization
for war, and actual combat. During
basic training, military accessions
come from disparate locations with
varying degrees of innate or
naturally acquired immunity, are
placed in relatively confined living
quarters and subjected to a high
degree of physical stress. As with
college freshmen in dormitories,
respiratory pathogens, in
particular, can spread easily in
this setting.
As sanitation and
personal hygiene improved,
airborne-transmitted infections
(e.g., mumps, measles, meningococcal
meningitis, influenza, pneumonia),
were recognized as the leading
causes of morbidity and mortality
among trainees. New chemotherapeutic
drugs, such as sulfonamides, became
available in the 1920s, but the need
for vaccines directed at primary
prevention was obvious. As new and
improved vaccines were developed,
each was considered for its
potential military value.
Influenza
During 1918-19, a
worldwide outbreak of viral
influenza killed 25 to 50 million
people, over 1% of the world
population. (3, 4, 6, 12, 13, 16,
43, 76-79) This pandemic caused the
greatest loss of life from any cause
in such a short period of time
throughout history. More than
500,000 deaths occurred in the U.S.
The global death toll was comparably
staggering. The world-wide pandemic
killed more Americans than the
military death tolls of all of
America’s wars in the 20th century
combined. Scientists tried a vaccine
against Pfeiffer’s bacillus (now
called
Haemophilus influenzae)
in the mistaken belief that the
epidemic had a bacterial cause, but
the effort naturally failed to quell
the tragedy. (80-82)
The first indication
of the developing American outbreak
came in March 1918 at Camp Funston,
Kansas, near present-day Fort Riley.
By April, cases appeared in most
American cities as well as among the
American Expeditionary Force under
General John Pershing, helping the
Allies repel the German Kaiser's
army from France. The hospital
commander at Camp Funston reported:
“There are 1,440 minutes in a day.
When I tell you there were 1,440
admissions in a day, you will
realize the strain put on our
Nursing and Medical force.” (78)
During those first
few months, the infection was
incapacitating, but not very lethal.
By August, however, virulence
increased and people increasingly
died. In response to the
incapacitation and deaths, theaters,
dance halls, bars, schools, and
other places of public assembly were
closed, including churches. Football
games were cancelled and telephone
booths were padlocked. The pandemic
weakened German military forces
perhaps more than Allied troops.
U.S. Army statistics
showed that 791,907 soldiers were
admitted to hospital in the U.S. or
France for influenza, and 24,664 of
them died. (83) Overall, one in 67
American soldiers died of influenza
or pneumonia in 1918. (4, 12, 43,
78, 79) Remembering how the outbreak
quickly sapped the fighting strength
of American troops, the U.S. Army
Surgeon General commissioned
research to develop influenza
vaccines in the 1940s, the first
iterations of the vaccines we still
use today. (1-4, 9, 12, 13, 84, 85)
Double-blinded field trials began in
U.S. service members, demonstrating
as much as 80% efficacy. In 1943,
the first influenza vaccine against
types A and B infection was used.
Subsequent military and civilian
vaccines contained at least one type
A and one type B component. In fall
1945 and spring 1946, all 7 million
troops were immunized against
influenza. Efficacy of all such
vaccines was dependent on
correlation of the vaccine’s
antigens with circulating viral
types, but scientists did not yet
fully appreciate the unceasing
antigenic variation of influenza
virus. The vaccine was not
considered effective in the
fall-winter 1947 season, because we
now know it was mismatched
antigenically. By the early 1950s,
annual immunization for all military
personnel was routine, a policy
continued ever since. (3, 7, 9,
86-88)
Assessments of
influenza vaccine containing various
mineral-oil adjuvants in the late
1940s and 1950s were promising, but
not pursued. Despite concerns about
the safety of the adjuvanted
vaccine, no prolonged morbidity or
mortality effects were evident two
decades after immunization. (3, 9,
89, 90) Memories of the 1918-19
influenza pandemic arose again when
an outbreak of severe respiratory
illness occurred in a basic training
camp at Fort Dix, New Jersey. Among
230 infected soldiers, 13 were
severely ill, with one fatality in
February 1976. (91-96) Laboratory
analysis showed the influenza virus
isolate to be one that had not
circulated among humans for more
than 50 years, and that was not
matched in the contemporary
influenza vaccine formulation.
Scientifically
designated A/New Jersey/76 (Hsw1N1),
the public called it “swine flu.”
This finding set in motion a process
that led to the National Influenza
Immunization Program, which DoD
participated in. (95, 96) In
November 1976, several cases of
Guillain-Barré syndrome (GBS), a
demyelinating neuromuscular
disorder, were reported from
Minnesota. Nationwide, 532 cases (32
fatal) were reported among vaccinees.
Epidemiologic investigation
suggested an increased risk among
civilian influenza vaccine
recipients that year, (97-100) but
not among military vaccine
recipients. (101)
One of the starkest
modern examples of the importance of
preventing influenza in military
communities comes from the USS
Arkansas, a cruiser sailing from its
homeport in February 1996. (102)
After influenza virus that did not
match strains used for immunization
entered the ship’s spaces, 42% of
the ship’s company became ill. The
rate of incapacitating illness was
sufficient to cause the ship to
cancel its training exercise and
make an unscheduled return to the
nearest port. Over the decades, the
benefits of influenza immunization
have become more apparent for
increasing sectors of the nation’s
population. Today, the most widely
used vaccine in America is influenza
vaccine, one of several vaccines
developed through military research.
Today, all active-duty members and
most personnel in the National Guard
or military reserves are immunized
annually, usually by the end of
December. Basic trainees receive
influenza vaccine through its
empiric June-30 expiration date each
year. The annually revised
composition of the influenza vaccine
is based on the prevalence of
strains of influenza A and B likely
to circulate, using the same vaccine
formulation adopted for civilian
personnel. To assist the global
health community in selecting the
most appropriate antigens for the
annual influenza vaccine
formulation, DoD sponsors an
extensive, global, laboratory-based
influenza surveillance program.
(103)
Meningococcal
disease
Meningococcal
meningitis is a life-threatening
bacterial infection that can spread
rapidly in dense populations. It
primarily is a disease of early
childhood and then wanes as
colonization in the gut and upper
airways with related bacteria
results in natural Immunity. A
secondary peak of disease incidence
occurs in early and late
adolescence, especially when
susceptible people come together, as
in military training camps,
colleges, and religious pilgrimages.
(3) It occurs with low frequency,
but a high case-fatality ratio.
Survivors may suffer brain damage,
learning disability, hearing loss,
or loss of limbs. The disease
occurred with disturbing frequency
in military trainees in the 1960s.
Antibiotic prophylaxis was used
initially, but the
Neisseria meningitidis
organisms became increasingly drug
resistant. In 1966, a meningococcal
research unit was organized at WRAIR.
(2-4, 6, 7, 12, 13, 104-107) The
first human tests of a vaccine to
protect against disease caused by
group C meningococci began in July
1967. In 1968, scientists led by
Goldschneider, Gotschlich, and
Artenstein at WRAIR developed a
serogroup C vaccine that prevented
disease and also reduced the
bacterial carrier rate. This was the
first modern polysaccharide vaccine.
Large clinical trials were
conducted in thousands of military
trainees. Their team defined the
humoral responses to the
meningococcal organism and the fact
that the subunit polysaccharide
vaccine could stimulate protective
immunity. Later, they developed its
serogroup A counterpart vaccine.
A few years later,
colleagues at the Institut Mérieux
in France manufactured similar
vaccines using the WRAIR
formulation. (7, 12) The work of
both teams permitted a massive
response to meningococcal serogroup
A epidemics that swept Finland and
Saõ Paulo, Brazil. In 1973, the
entire population of Finland, over 4
million people, was immunized
against group A at a series of mass
immunization clinics to control an
epidemic. The Brazilian epidemic of
1974 produced 150,000 cases of
meningococcal disease and 11,000
deaths. In one of the most
dramatic mass immunization efforts
ever, 100 million doses of serogroup
A vaccine were administered during
the Brazilian epidemic. These
and successor vaccines are now used
to prevent disease outbreaks among
military trainees and in other
settings. (2-4, 12, 107)
Widespread use of
meningococcal A/C vaccine among U.S.
military trainees began in 1971.
Along with reducing trainee class
sizes, immunization reduced the risk
of fatal meningococcal disease
during basic training. (2-4, 12,
107) Meningococcal immunization has
been part of the core immunization
requirement for new trainees ever
since. Sanofi Pasteur’s tetravalent
vaccine against serogroups A, C, Y,
and W-135 ( Menomune)
received a U.S. license in January
1978. (7) The military success with
meningococcal immunization among
repeated iterations of
Immunization to
Protect the U.S. Armed Forces
newly assembled cohorts was cited
when recognition of elevated rates
of meningococcal disease among
college freshman and dormitory
residents led to calls for
immunization in those populations
since the late 1990s. (108)
Meningococcal
immunization marked another advance
in January 2005 when the FDA
licensed Sanofi Pasteur’s
protein-conjugated meningococcal
vaccine,
Menactra.
Its proteinconjugated
characteristics may offer prolonged
duration of immunity, compared to
polysaccharide immunization. (7)
Unfortunately, attempts to develop a
serogroup B meningococcal vaccine
have not yet been fruitful.
Adenoviruses
After World War II,
adenovirus infections (particularly
serotypes 4 and 7) were noted to
infect up to 80% of military
trainees and were linked with
epidemic acute respiratory disease (ARD)
outbreaks in training camps. (3,
109-118) Up to 60% of trainee ARD
resulting in hospitalization was
linked to adenovirus infections.
Infections in seasoned military
personnel were less frequent. Before
widespread immunization of trainees,
600 to 800 acute respiratory-disease
hospitalizations per week occurred
at military basic-training sites in
the northern United States in the
early 1960s, disabling 40 to 50% of
these closed communities. Adenovirus
infection, which resembles influenza
in clinical manifestations,
represented the leading cause of
military hospitalizations in the
United States at that time.
Hospitalization rates of 6 to 8% per
week typically occurred during basic
training cycles.
In 1956, WRAIR
developed formalin-inactivated
vaccines against adenovirus types 4
and 7. (2-4, 6, 7, 12, 88, 109-119)
Such vaccines were marketed by
Parke-Davis as "common cold"
vaccines from 1957 to 1965, with
viral types 3, 4, and 7 represented
in the vaccine. Maurice Hilleman’s
team at WRAIR demonstrated in 1958
that adenovirus vaccines of types 4,
and 7 reduced adenovirus disease
incidence by 60 to 90% among U.S.
soldiers under the stressful and
crowded conditions of basic
training, with cross protection
against type 3. A report estimated
that the vaccine saved the Army
about $5 million a year in 1973
dollars. (99)
Beginning in 1959,
inactivated adenovirus and influenza
virus antigens were combined in a
Parke-Davis product known as
Resprogen.
(7) Several million doses were sold
between 1959 and 1965. In
retrospect, the need to make annual
adjustments to influenza vaccine
antigens made this combined product
irrational. But the need to change
influenza viral antigens annually
was not fully recognized until the
early to mid-1960s. In 1963, viral
seed lots for this vaccine were
found to contain the oncogenic SV40
virus as well as SV40 genome in the
adenovirus capsids. (31, 120-122)
Safety concerns and lack of efficacy
caused the product to be withdrawn
from distribution. Several studies
have shown no elevated risk of
cancer in these vaccine recipients.
The live types 4 and 7 adenoviruses
used in the modern products have
been shown not to be oncogenic.
(123)
In 1964, clinical
trials of live, attenuated type 4
vaccine began at WRAIR. (2-4, 6, 7,
12, 113, 114, 116-118, 124) Trials
of type 7 begin in 1969 and type 21
in 1971. Type 7 adenovirus vaccine
was added to the regimen given
American military trainees in 1970.
These vaccines were developed as
oral tablets in the 1970s and
licensed in July 1980. The tablets
were given shortly after arrival at
a basic training center, protecting
within a few weeks after
administration. (87) Adenovirus
vaccines produced dramatic
reductions in disease incidence.
Immunization induces specific
protective serum and secretory-intestinal
antibodies, protecting against
infection for at least 60 days and
presumably longer.
By 1984, both
vaccines were routinely administered
as tablets to trainees at all basic
training camps year-round.
Unfortunately, the manufacturer (Wyeth
Laboratories) and DoD did not make
capital improvements to the
manufacturing facilities for these
vaccines, and production ceased in
1996. (10) The last lots of these
vaccines expired in 1998. Since
then, disease outbreaks among
trainee populations have recurred,
including several deaths. (123,
125-128) A replacement manufacturing
line for adenovirus type 4 and type
7 vaccines will be submitted for
Immunization to Protect the U.S.
Armed Forces
regulatory review. (10, 129, 130)
Measles, Mumps,
Rubella, Varicella
During the
Revolutionary and Civil Wars,
measles was one of the principle
causes of death among troops.
Measles and secondary pneumonias in
1917 led to 48,000 hospitalizations
and 1 million lost work days, and
represented 30% of all Army deaths.
(43, 131, 132) During 1917 and 1918,
mumps was a leading cause of days
lost from active service by members
of the American Expeditionary Force
in Europe. (16, 43, 133) During
World War II, measles, mumps,
rubella, and varicella accounted for
over 300,000 admissions to hospital
or restrictions to quarters.
(134-135) In 1961, Paul Parkman and
colleagues at WRAIR were
co-discoverers of the rubella virus,
isolating the virus among trainees
at Fort Dix. (3, 135) Even into the
1970s, measles and rubella caused a
substantial number of
hospitalizations and lost training
time at basic training centers. (87)
Vaccines to prevent
measles, mumps, and rubella were
licensed in the U.S. between 1963
and 1969. The AFEB helped fund
development of an attenuated measles
vaccine. (136) For military
trainees, rubella vaccine was
adopted first, in 1972, with measles
vaccine added in 1980, to immunize
those who avoided infection as
children. (9, 137, 138) Mumps
outbreaks were less common than the
other two diseases, so mumps
immunization was not uniformly
adopted until 1991. (10, 139) A
varicella policy of screening and
as-needed immunization was also
adopted in 1991. (10) The FDA
licensed varicella vaccine in 1995.
Now that a large proportion of basic
trainees enter military service
immune to these infections, due to
childhood immunization, the Services
are testing for antibody and
exempting those already immune. (10,
140-146)
Diphtheria
Diphtheria toxoid
was first licensed in the United
States in 1926 and was later
combined with tetanus toxoid to
simplify the task of injecting the
two products. But diphtheria toxoid
was known to cause substantial
injection-site swelling if injected
into someone already immune to
diphtheria. So diphtheria
immunization policy was complicated
by whether or not to perform the
Schick skin test to determine if an
individual was immune (induration
after injecting diphtheria toxin is
considered a positive test, implying
susceptibility). (7, 14, 147, 148)
Military clinicians noted the
injection-site swelling that
followed diphtheria toxoid
administration in adults and
developed a reduced-dose
formulation. The work of Geoffrey
Edsall and colleagues at the Great
Lakes Naval Training Center in the
1950s demonstrated that this
approach was comparably immunogenic,
but with fewer injection-site
symptoms. (3, 7, 149, 150)
The practice of
administering DT (full strengths of
both diphtheria and tetanus toxoids)
to children up to the seventh
birthday and Td (full-strength
tetanus and reduced-strength
diphtheria toxoid) to older children
and adults continues to this day.
(7) PROPHYLAXIS OF DISEASES WITH
ECOLOGIC NICHES Deployment vaccines
include those vaccines administered
to personnel sent to regions where
the risk of contracting a specific
endemic vaccine-preventable disease
is increased. Deployment vaccines
include typhoid, hepatitis B,
meningococcal, yellow fever,
Japanese encephalitis, and rabies.
The first few were discussed above.
Beyond its use in military basic
training, meningococcal immunization
may be required for specific
assignments (e.g., personnel
traveling to sub-Saharan Africa
during the dry season (December to
June)). (66)
Yellow fever
Yellow fever was a
significant problem for U.S. troops
throughout the southern United
States in the 19 th
century. This infection was
especially troublesome during the
Spanish-American
Immunization to Protect the U.S.
Armed Forces
War of
1898, particularly in Cuba. (2-4, 6,
12, 13, 16, 31, 151, 152) To
investigate, Army Surgeon General
Sternberg appointed another board of
investigation. Walter Reed and his
colleagues proved Carlos Finlay’s
hypothesis of transmission of the
disease by mosquito. (153-157) The
follow-up research ultimately led to
the isolation of the virus.
Separately, Max Thieler attenuated
yellow-fever virus by serial cell
culture passage in 1927. The
resulting vaccine strain 17D is
still used today for travelers to
yellow fever-endemic areas of the
world, including deployed military
personnel.
During World War II,
yellow fever was considered both a
natural threat, with speculation
about its use as a biologic weapon
if adversaries could release
infected mosquitoes. (158) As a
result, a yellow-fever immunization
program was instituted for selected
personnel in the U.S. Armed Forces.
By April 1942, 7 million doses of
the vaccine had been given. The
program was complicated by reports
of hepatitis in recipients. (2-4,
12, 54, 56, 68, 69) In March 1942,
100 cases of jaundice and hepatitis
were noted at training camps in
California, soon after yellow-fever
immunization. Health authorities
quickly realized that the diluent
for yellow-fever vaccine contained
human serum albumin that had not
been heat treated. The albumin was
contaminated with a previously
unrecognized virus that caused
hepatitis (i.e., hepatitis B virus).
Immunizations ceased and the
Rockefeller Foundation stopped
producing the serum-containing
product midway through 1942, until
it could develop a serum-free
formulation. By December 1942, over
50,000 cases of hepatitis B and 84
deaths followed some 2.5 million
yellow-fever immunizations from
certain lots. This accident helped
reveal the differences between
hepatitis A (then called “infectious
hepatitis”) and the newly recognized
hepatitis B virus (“serum
hepatitis”). This incident
highlights the risks of using
vaccines in large populations
without preliminary safety testing,
now required before licensure of any
vaccine.
In 1985, follow-up
studies interviewed and sero-screened
597 Army veterans from 1942. The
authors concluded that hepatitis B
virus caused the outbreak, that
about 330,000 persons may have been
infected, that the hepatitis B virus
carrier state was a rare
consequence, and that the outbreak
induced hepatitis B-specific
antibodies that appear to persist
for life. (159) The small excess
liver cancer mortality seen in a
related cohort study and the results
of a case-control study are
consistent, nevertheless, with the
now well-established etiological
role of hepatitis B virus inliver
cancer. (160) In addition to the
hepatitis-B issue, the yellow-fever
vaccine of the 1940s was grown in
eggs of chickens infected with avian
leucosis virus. Evaluation
identified no related harm in
vaccine recipients. (161)
From the 1950s
onward, yellow-fever immunization
for adults proceeded with few
problems, until recently.
Yellow-fever vaccine is a live
attenuated product given to
personnel with assignments to yellow
fever-endemic areas. As a general
rule, all personnel assigned to
Latin America or sub-Saharan Africa
or with missions that may take them
to these regions are immunized. But
recent concerns about rare cases of
yellow-fever vaccine-associated
neurotropic disease raise questions
about whether military forces should
use the vaccine narrowly (i.e.,
focused on those traveling soon), or
broadly (i.e., to those who may
travel eventually, to minimize the
number of immunizations given just
before departure). Military policy
makers, in concert with medical
consultants, work to balance the two
competing objectives. (10, 162) The
goal is to optimize benefit and
minimize risk for service members.
Japanese
encephalitis
A Japanese
encephalitis (JE) vaccine was
produced in 1943 through efforts of
Albert Sabin and colleagues in the
Far Eastern theater, based on a
Russian vaccine. JE vaccine was
administered to a limited number of
personnel in Japan (primarily
Okinawa) in 1945 during an outbreak
in the civilian population. (4, 12,
16, 163, 164) This vaccine was
derived from virus infected mouse
brain.
Immunization to Protect the U.S.
Armed Forces
By the 1980s,
military, diplomatic, and travelers’
need for a JE vaccine led to studies
led by military scientists that
permitted U.S. licensure of JE
vaccine. (7, 165-168) The FDA
licensed JE vaccine in 1992. Because
of an uncommon risk of delayed-onset
urticaria and angioedema, the
vaccine’s labeling recommends
deferring travel for 7 to 10 days
after immunization. Current military
policy provides this vaccine to
military members on assignments to
areas with a high endemnicity of
disease, primarily on Okinawa with
extended field exposure and rural
areas of Thailand. The manufacturer
intends to cease production in mouse
brains and transition to a cell
culture production process.
Rabies
Today, pre-exposure
rabies vaccine is provided to
selected troops who have missions to
areas endemic for rabies and who may
have an elevated risk of being
bitten by a rabid animal. The
rationale for immunization derives
from a lack of ready access to
definitive medical care if bitten.
In addition, veterinarians,
veterinary technicians, and those
with animal-control responsibilities
receive standard occupational
pre-exposure immunization.
Prophylaxis policies follow ACIP
guidelines. (7, 30, 168, 169)
PROPHYLAXIS AGAINST
BIOWEAPONS
Smallpox
The historic use of
smallpox vaccine was addressed
above. In December 2002, a national
program of smallpox vaccination
resumed, to counter the consequences
of a malicious release of variola
virus. (28) A smallpox outbreak
would significantly affect military
readiness. An outbreak would degrade
combat-mission capability among
vulnerable troops; stress military
medical operations to maximum
capacity; restrict military
operations; limit transit of
international boundaries; and divert
military manpower for health care or
crowd control.
In a remarkable
example of mass individualized
immunization, more than 400,000
service members deploying to
southwest Asia were screened for
smallpox immunization. (28) After a
trial run at Walter Reed Army
Medical Center, military clinics
used standardized education
materials, concise screening forms,
bandages, and staff training to
educate recipients about the
idiosyncrasies of smallpox
vaccination, identify
contraindications (e.g., atopic
dermatitis), safely administer the
vaccine, and care for the
vaccination site appropriately. The
accompanying prospective
surveillance system identified an
elevated risk of myopericarditis in
the second week after primary
smallpox vaccination, especially
among young adult Caucasian men.
(170-172) The military smallpox
vaccination program continues, to
preserve critical military
capabilities in case of an attack,
with over 1,020,000 people screened
and over 940,000 people vaccinated
between December 2002 and January
2006.
Anthrax
The intelligence
community and civilian experts
consistently rank anthrax spores as
the number one threat from
bioweapons. This ranking is due to
the stability of spores, which can
persist for decades despite
environmental extremes. Anthrax
spores can be easily dispersed, as
seen in the multiple releases via
mailed letters or packages on the
U.S. eastern seaboard in fall 2001.
(173, 174)
Early anthrax
vaccines were developed at Fort
Detrick, Maryland, by George Wright
and colleagues. (175-180) Anthrax
vaccine adsorbed was tested in a
human field trial in the 1950s,
demonstrating 92.5% reduction in
disease incidence (cutaneous and
inhalation cases combined), (181)
and licensed in 1970. (182, 183)
Subsequent inhalation challenges in
nonhuman primates and rabbits showed
greater than 95% protection against
lethal challenge. A comprehensive
review by the National Academy of
Sciences (NAS) affirmed the efficacy
of anthrax vaccine adsorbed. (179)
An estimated 150,000
American troops received one or two
anthrax immunizations during the
Persian Gulf War in 1991, but
individual records were either not
kept (in an attempt not to identify
those unvaccinated, hence vulnerable
to enemy bioweapons) or were marked
with terms such as “Vaccine A.”
(179, 184-188) In 1998, a much
larger immunization program began
that has now administered over 5.6
million anthrax immunizations to
over 1.4 million troops. Anthrax
immunizations are primarily intended
for people serving in areas judged
to be at higher risk (e.g.,
southwest Asia, Korea), as well as
military personnel with homeland
biodefense roles. Anthrax vaccine
was the target of prolonged
skepticism, evoking the NAS review
and an extraordinary array of
post-marketing safety studies. These
studies involved cohort studies of
acute symptoms, (189-197)
hospitalizations, (179, 198)
disability evaluations, (199) and
reproductive outcomes, (200-202) as
well as secondary review of the
spontaneous reports to the Vaccine
Adverse Event Reporting System (VAERS).
(203, 204) Anthrax-vaccinated and
unvaccinated personnel had
comparable rates of illness and
health. Several of the cohort
studies span observation for
multiple years after immunization.
(179, 198, 199, 201, 205, 206)
After the National
Academy of Sciences heard from
vaccinees and comprehensively
reviewed the accumulated scientific
data, it concluded that anthrax
vaccine has an adversereaction
profile similar to other adult
vaccines. (179) The AFEB concurred.
(10) However, an elevated rate of
injection-site pain and swelling,
occasionally with peripheral
neuropathy, is associated with
administering a vaccine adjuvanted
with aluminum hydroxide by
subcutaneous injection. Studies
underway are evaluating rare adverse
events (e.g., prolonged myalgia/arthralgia)
and the relative effectiveness of
intramuscular injection on
immunogenicity and safety. The
anthrax immunization program also
pointed out needed improvements in
the way DoD exchanges information
with military personnel and their
families and provides clinical
immunization services in general.
Plague
A
formalin-inactivated plague vaccine
saw limited use during World War II.
(3, 15, 180) American soldiers
deploying to Vietnam during the
1960s received a similar inactivated
plague vaccine. Conversely, the
South Vietnamese government gave the
live EV or EV76 strain of plague
vaccine to its soldiers. (2, 3, 18,
180, 207-211) American use of plague
vaccine declined substantially after
the 1960s, given a relatively high
degree of injection-site reactions
and limited exposure to the
bacteria. Today, no plague vaccine
licensed by the Food & Drug
Administration is manufactured. (7,
10, 130, 212)
A plague vaccine is
potentially of interest in
countering bioweapon threats, (213)
but that whole-cell plague vaccine
did not adequately protect mice
against inhalation challenge with
Yersinia pestis bacteria. (214)
Modern technology may provide an
improved plague vaccine containing
F1 and V proteins as the principal
antigens. (210, 211, 215)
PROPHYLAXIS OF OTHER
INFECTIOUS HAZARDS
Pneumococcal Disease
Early tests of
pneumococcal polysaccharide vaccines
occurred at Camp Upton, New York,
and Camp Wheeler, Georgia, in
1918-19. During the 1930s,
polyvalent pneumococcal
polysaccharide vaccine was tested in
five trials in 120,000 men at
Civilian Conservation Corps camps.
(4, 7, 12, 13, 16, 216-219) In 1937,
Frank Horsfall prepared a
therapeutic rabbit pneumococcal
antisera. It was less reactogenic
than a similar product prepared with
equine serum. Equine or rabbit
pneumococcal antiserum was available
from several sources as late as
1965. (4, 7)
Successful clinical
trials of pneumococcal vaccine were
conducted in military trainees in
1944-45 at Sioux Falls Army Air
Force Technical School experiencing
a high incidence rate of
pneumococcal infections. (2-4, 12,
218, 219) Based on this work, E. R.
Squibb & Son marketed two hexavalent
pneumococcal polysaccharide
vaccines, in either adult or
pediatric formulations. These
vaccines were not widely prescribed
because of greater confidence in
another newly introduced drug,
penicillin. The vaccines were
voluntarily withdrawn by the
manufacturer in 1954, due to lack of
acceptance and low sales. Subsequent
studies showed that penicillin did
not alter the death rates of
pnuemococcal disease during the
first four days of infection,
renewing interest in prevention by
immunization. Today, pneumococcal
polysaccharide vaccine 23-valent is
given to asplenic military
personnel. Based on episodic
outbreaks, the vaccine has also been
given to selected Marine Corps and
special operations trainees; its
value in training settings is being
evaluated. (10, 220)
Poliovirus
The development of
poliovirus vaccines can be traced to
the AFEB in the 1940s. Jonas Salk
was charged with developing a typing
system for polioviruses and Albert
Sabin was charged with developing an
attenuated vaccine. In 1955, the
U.S. government licensed Jonas
Salk’s inactivated poliovirus
vaccine. In 1961, the first of
several formulations of Albert
Sabin’s oral attenuated vaccine was
licensed, and 1 year later, the oral
polio vaccine largely replaced the
Salk vaccine in the U.S. (7, 18)
Initially, military poliovirus
immunization was a “catch-up”
program for adults who hadn’t been
immunized as children. Basic
training centers switched from
injectable vaccine to oral vaccine
once the trivalent oral product
became available in the early 1960s.
(9) DoD policies have been revised
over time as the global incidence of
poliomyelitis has declined. Today,
the Services administer one dose of
inactivated poliovirus vaccine to
their trainees, consistent with the
ACIP recommendation for a single
adult dose (after the basic
immunizing series) for international
travelers. (10, 221)
Historical Notes
Regrettably, the
content above is not an exhaustive
description of the details of the
American experience. But several
vaccines given to earlier
generations of American troops, but
no longer used, are worthy of brief
mention. The military immunization
experience includes long-term
follow-up of laboratory personnel
who received multiple common and
exotic vaccines. (205, 222- 227)
A
formalin-inactivated typhus vaccine
was primarily provided to troops
serving in Europe during World War
II and then in the Korean and
Vietnam wars. Plotz and colleagues
at WRAIR helped purify the specific
antigen. The vaccine prevented
louse-borne (epidemic) typhus, not
murine or scrub typhus. The microbes
were cultured in chicken-embryo yolk
sacs and inactivated with
formaldehyde, the Cox method, and
first licensed for general use in
1941. (2-4, 7, 9, 228-231) However,
subsequent attempts to purify this
vaccine resulted in inadequate
potency, so immunization eventually
gave way to insecticides and
antibiotics. Production voluntarily
ceased in 1980 and the last batch
expired in 1981. During the late
1980s, an inactivated tick-borne
encephalitis vaccine produced in
Austria was administered as an
investigational vaccine to certain
inspectors enforcing the
Intermediate- Range Nuclear Forces
Treaty. (10, 130) These inspectors
regularly visited rural and forested
areas of the Soviet Union that are
highly endemic for tick-borne
encephalitis. A similar product was
used in 1996 during U.S. military
deployment to Bosnia. (7, 10, 130,
168, 232, 233)
In the 1950s, IGIM
was used to treat patients deficient
in the antibody-rich gamma-globulin
fraction of serum, first described
by COL Ogden C. Bruton, an Army
pediatrician at Walter Reed Army
Hospital. (234-236) His discovery
opened new approaches in passive
immunization and the diagnosis and
treatment of humoral (antibody)
immune deficiencies. Human
hyperimmune globulins largely
replaced corresponding equine
antisera and antitoxins in the
1960s. (7, 9, 46)
VACCINE-SAFETY
SURVEILLANCE PROGRAMS
With the success of
immunization in reducing the
incidence of diseases discussed
above, the military health system
faces the same challenges that the
civilian public-health sector does –
increasing concerns about vaccine
safety and adverse events
experienced after immunization. Even
one adverse event among thousands of
vaccine recipients, if serious or
with prolonged health impact, can
cause concerns about the safety of
an immunization program. Vocal
objection to military immunization
programs occurred with variolation
in the 1770s, (6, 16) smallpox and
typhoid vaccines in the 1910s, (27,
37) various vaccines in World War
II, (14) and anthrax vaccine in the
1990s. (179) Few conditions are
uniquely caused by immunization. One
of the few examples is paralytic
poliomyelitis that rarely follows
use of the live attenuated
poliovirus vaccine. Instead,
immunizations can be risk factors
that increase the relative risk of
an adverse event occurring (e.g.,
Guillain-Barré syndrome that was
more likely with some annual
formulations, but not others, of
influenza vaccine). On the other
hand, health conditions that occur
in unvaccinated people are fully
expected to occur in vaccinated
people, at the same background rates
of incidence. Discerning when an
adverse event that occurs after
immunization is an adverse reaction
that should be causally attributed
to immunization can be a clinical
challenge. In addition, the
important task of explaining
benefit-risk ratios for individual
patients requires time, experience,
and training. The military health
system that implements immunization
programs also has a responsibility
to implement safety surveillance
programs. (237) In recent times,
these surveillance programs may be
best exemplified by assessments of
anthrax vaccine safety and smallpox
vaccine safety, where the U.S. DoD
has been the primary user of these
vaccines. (28, 170-172, 179,
189-206) A Navy allergist was among
the first to recognize the role of
gelatin in vaccine-associated
anaphylaxis. (237)
PRACTICAL ISSUES IN
PROGRAM IMPLEMENTATION
Most vaccines
require continuous refrigeration. A
few require storage in a freezer.
Maintaining the “cold chain” to
assure injection of potent vaccines
appears simple, but requires
considerable effort. (7, 14-17) In
World War II, smallpox vaccine was
transported by air using
kerosene-powered refrigerators or
packed in dry ice. Improper vaccine
storage was one of the principle
factors in breakthrough infections.
In January 1946, a special shipment
of smallpox vaccine was ordered from
the U.S. mainland, after doubts
arose about the potency of vaccine
on the Korean peninsula. (26, 238)
Today, monitoring devices can record
the temperature of vaccine
shipments, allowing improperly
handled product to be discarded
rather than injected. Documentation
of immunizations is important to
record a healthcare encounter and to
avoid redundant immunization at
future healthcare visits. During
World War II, tetanus immunizations
were marked on troops’
identification tags (“dog tags,”
Figure 3) and on paper records
(Figures 1 and 2). Increasingly
since in the 1990s, DoD uses
electronic immunization tracking
systems (i.e., registries) to
permanently record immunizations,
even if paper records are lost. (10,
239)
In civilian adult
immunization programs, individuals
can choose to accept or decline
authoritative recommendations to be
immunized. In military settings,
mission-oriented teams place an
extraordinary reliance on the health
of each team member. The
interdependence of members of a
military unit has vital consequences
in military settings, where the loss
of one service member from an
infectious disease could degrade
unit performance and cause the loss
of other service members due to
enemy action. This interdependence
leads to command policies requiring
military immunizations. The unity of
command inherent to military
organizations allows public-health
decisions to be applied consistently
across dispersed military
communities.
The administration
of immunizations to new military
personnel is based on several
assumptions. First, entrance
physical examinations establish that
trainees comprise a healthy
population without underlying
conditions known to predispose
people to serious adverse effects
from immunization (e.g., immune
deficiencies). Second, most trainees
are assumed to have been exposed to
childhood vaccine antigens through
natural infection or childhood
immunization programs. Vaccines with
highest priority are those to
prevent infections most
transmissible in closed settings
(e.g., meningococcal disease,
influenza, measles, varicella). (8)
During training,
accessions must acquire immunity to
multiple infections within a short
period of time. Thus, simultaneous
immunizations have been provided to
tens of millions of trainees over
the last 65 years. (9, 10, 223, 239)
The AFEB considered the issue and
found that available data “do not
demonstrate serious or long-term
adverse health effects causally
related to multiple, concurrent
immunizations, and there is no
reason to deviate from current
consensus guidelines for adult
immunization.” (240) Similarly, an
Institute of Medicine committee and
others found the evidence favors
rejecting a conclusion that
simultaneous immunization causes
heterologous infection, type-1
diabetes, or other patterns of
adverse events. (241, 242)
Additional work is needed to
identify risk factors that might
predispose to rare problems. Because
American adolescents today have a
high degree of pre-existing
immunity, the DoD increasingly uses
sero-screening to individualize
immunizations according to personal
vulnerabilities. (10, 140-146,
243-246) Training sites are planning
to separate immunizations into
clusters based on acute versus
long-range need. The first cluster
would protect against pathogens
posing an imminent risk in closed
communities (e.g., influenza;
meningococcal; measles, mumps,
rubella; varicella). The second
cluster would protect against
pathogens posing a threat later in
military service (e.g., hepatitis A,
hepatitis B, poliovirus,
tetanus-diphtheria). Additional
vaccines can be given later during
training. The Marine Corps, for
example, administers yellow fever
vaccine to trainees late in the
training cycle. Typhoid or rabies
immunization can be started during
advanced or specialty training,
based on first assignment or
occupational specialty,
respectively. (8)
Jet-injection
devices were first developed in the
private sector in the 1940s.
Military scientists adapted the
early devices to create needle-free
multi-use-nozzle jet injectors
capable of 600 or more subcutaneous
injections per hour from 1949
onward, primarily for basic training
camps. The Army’s Aaron Ismach and
Abram Benenson developed a nozzle
for intradermal vaccination, used in
civilian mass smallpox immunization
campaigns in the 1960s. (3, 9, 19,
247- 251) Unfortunately, the
devices’ use of the same unsterile
nozzle and fluid pathway to inject
consecutive patients allowed
transmission of blood-borne
pathogens (e.g., hepatitis B, human
immunodeficiency virus) in civilian
settings, and the devices have
fallen into disfavor. (252, 253) In
contrast, a new generation of
disposable-cartridge jet injectors
is being developed to avoid these
safety concerns by using a
disposable, sterile fluid pathway
for each patient. These devices have
not yet been widely adopted for
military use because of relatively
slow handling characteristics and
high unit costs. But research is
underway for automated prefilling
and finger-free loading and ejecting
of cartridges, to make future
high-speed jet injectors suitable
for mass-immunization programs.
RESEARCH PORTFOLIO
Military personnel
confront infectious hazards for
which there are not yet licensed
vaccines. Over the past few decades,
the U.S. military medical research
community, working with civilian
partners, developed several
investigational vaccines, toxoids,
and immune globulins, to counter
specific military threats associated
with high morbidity. These threats
included natural, endemic diseases,
and microbes that could be deployed
as bioweapons. (130, 178) Such
vaccines have been studied to
prevent Argentine hemorrhagic fever
(AHF, Junín virus), (152, 254, 255)
botulism, (256) Chikungunya, (152,
257, 258) dengue fever, (254, 259)
eastern equine encephalitis (EEE),
(260) Ebola fever, (152, 254, 261,
262) Escherichia coli, (33, 263)
hepatitis E, (264) HIV disease,
(265-268) Lassa fever, (152, 254,
261), malaria, (269-272) Mycoplasma
pneumoniae, (273) Neisseria
meningitidis serogroup B, (274,
275) Q fever, (180, 276, 277) Rift
Valley fever, (152, 254, 278-282)
shigellosis, (33, 283) tularemia,
(180, 227, 284-287) Venezuelan
equine encephalitis (VEE), (2, 168,
227, 288-290), and western equine
encephaltitis (WEE). (291) A
gonococcal vaccine produced
neutralizing antibodies, but was not
sufficiently protective in a field
trial. (268, 292)
New versions of some
of the older vaccines are in early
clinical trials, including
recombinant botulinum bivalent AB
vaccine and live attenuated VEE
V3526 vaccine. (293) Next-generation
anthrax vaccines in clinical trials
include recombinant protective
antigen vaccine and a vaccine
adjuvanted with CpG
oliogdoxynucleotides. (294-296) The
role of anthrax capsule/exosporium
and toxin proteins and spores are
being evaluated in animal models. A
F1-V plague vaccine should enter
clinical trials soon. (215) Vaccines
advancing toward clinical trials
include staphylococcal enterotoxin
B/A vaccine, (297) Hantavirus
vaccine, (152, 298, 299) and
next-generation EEE (168, 289, 300)
and WEE vaccines. (168, 289, 300)
Novel approaches being pursued
include RNA replicons as vaccines
against Marburg and other
filoviruses, (301, 302) and DNA
vaccines against Hantavirus. (303)
The candidate malaria vaccine RTS,S
reduced the rate of parasitemia by
37% and severe malaria by 58% over a
6-month period in Mozambiquean
children. (270, 271) Like the
movement toward more combination
vaccines for children, multiagent
vaccines for military personnel are
also a priority. A panfilovirus
vaccine protecting against Marburg
and Ebola variants might be possible
within a decade. Novel delivery
systems being pursued include
aerosols, micro-needle or adjuvanted
patches, and oral dosage forms.
Similarly, human
immune globulin preparations (e.g.,
hyperimmune globulins, antitoxins)
have been investigated to treat or
protect against Klebsiella and
Pseudomonas infections in burned or
severely wounded troops, (304) and
to provide passive protection
against Lassa fever, (152, 254),
Ebola fever, (254, 305) Bolivian
hemorrhagic fever (caused by Machupo
virus), (152, 306) and botulism
(307). Military researchers
established the clinical value of
IgG antibodies to prevent
respiratory syncytial virus. (7,
133, 308) Polyclonal and monoclonal
antibodies are being evaluated for
treatment of anthrax infection. VEE
vaccines were given to 2 million
horses in Texas to control an equine
outbreak there that had contributed
to 84 human deaths. (2, 168) The
formalin-inactivated Rift Valley
vaccine was given to 963 Swedish
soldiers under the United Nations’
peace-keeping flag in the Sinai
Peninsula during the outbreak until
1979. (281, 280, 309, 310) More than
175,000 doses of the AHF vaccine
have been administered in Argentina,
dramatically reducing disease
incidence. (152) Even successful
vaccine research may not be carried
through to commercial development
and licensure, such as improved
vaccines against Rocky Mountain
spotted fever developed at WRAIR.
(231, 311, 312) About 8,000 troops
received investigational botulinum
toxoid ABCDE during the Persian Gulf
War. (184-188)
Military personnel
need vaccines that are: (1) safe
(associated with few adverse
reactions), (2) effective (able to
produce long-lasting immunity), (3)
easy to administer, (4) durable
(prolonged shelf life, tolerant of
shipping conditions), and (5)
affordable (offering good value for
the price paid). The ideal vaccine
formulation would produce immunity
against several diseases through a
single dose. Genetically engineered
products may lend themselves to this
ideal. Adjuvant research will help.
(313) Yet even with 20 years
experience in biotechnology, the
development and licensing process is
no shorter or simpler than it was in
the 1980s. Even so, DoD invests in
the research and development of
additional countermeasures to
natural infectious diseases and
bioweapons that might harm military
personnel. In addition, DoD will
take advantage of the fruits of
private-sector research and
development. The private-sector
pipeline may soon deliver vaccines
to prevent papilloma virus-related
cervical cancer, rotavirus
gastroenteritis, and herpes zoster.
SUMMARY
In 1900, smallpox
vaccine was widely used, rabies
vaccine was available as
post-exposure prophylaxis after
animal bites, and typhoid vaccine
was just coming to public attention.
A century later, 21 serious
infections can be prevented with
FDA-licensed vaccines. (6-8) As
vaccines increase in number and
national focus on vaccine safety
continues, the complexity of
managing clinical immunization
challenges will undoubtedly
increase. Information technologies
are increasingly used to disseminate
immunization information (e.g.,
www.vaccines.mil) and conduct
long-distance, on-demand training
(e.g.,
www.projectimmunereadiness.amedd.army.mil).
Today, military units in the United
States conduct immunization programs
at training camps, before overseas
deployments, and annually during
influenza immunization campaigns.
The goal is to protect individual
health and to keep units strong so
they can accomplish their military
missions. The FDA-licensed vaccines
selected protect against infections
during training itself, as well as
during subsequent years. The
vaccines of most acute need during
military training protect against
pathogens that represent an imminent
risk of contagious disease in
settings of close contact. Other
vaccines are given to prevent
infections more likely to occur
later, during international travel
or during extended periods of
military service when poor
sanitation or bioweapons could
threaten their lives.
Military
immunization programs maintain the
health of soldiers, marines,
sailors, airmen, and coast
guardsmen, the most important
resources within the Department of
Defense, the resources most critical
to military success. These people
deserve the best available
protective measures. Some
immunization needs are universal for
all in military service (e.g.,
tetanus) while others derive from
specific environmental or
occupational risks (e.g., Japanese
encephalitis, rabies). But military
immunization programs need to be
individualized based on personal
contraindications or prior immunity.
The proper conduct of military
immunization programs respects the
need for detailed education of
military personnel about the
immunizations they get, maximizes
quality in immunization delivery,
and supports quality clinical care
to prevent and treat adverse events
after immunization.
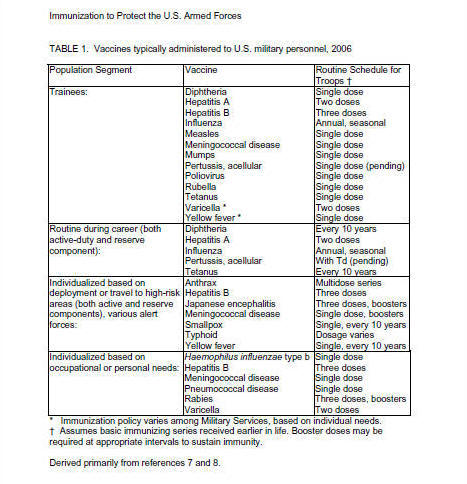
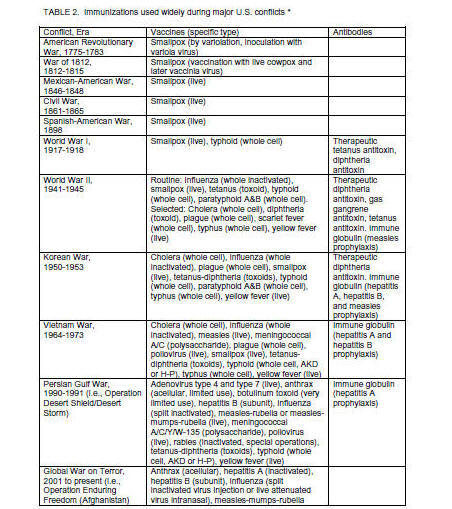
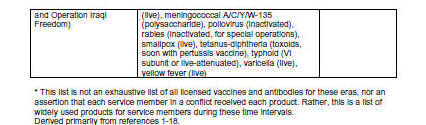
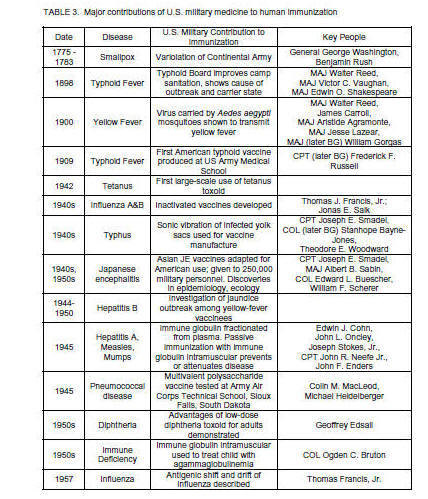
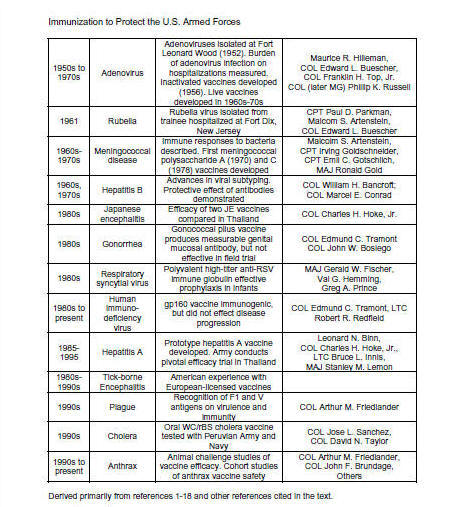
Acknowledgements
The critical
comments on early versions of this
manuscript by COL (Ret) Leonard N.
Binn, MD; LTC Stephen M. Ford,
PharmD; COL (Ret) Joel C. Gaydos,
MD, MPH; COL (Ret) Frederick E.
Gerber, MS; COL (Ret) Charles H.
Hoke, Jr., MD; COL (Ret) Richard N.
Miller, MD, MPH; COL (Ret) Ernest T.
Takafuji, MD, MPH; COL Gaston M.
Randolph, Jr., MS; MG (Ret) Philip
K. Russell, MD; COL (Ret) Edmund C.
Tramont, MD; and CAPT Bruce G.
Weniger, MD, MPH, are gratefully
acknowledged. We also recognize the
library-science skills of Yevetta
White, Emily A. Court, Tom
Cunningham, and Blythe Carey
enhanced this manuscript.
Abbreviations
ACIP - Advisory
Committee on Immunization Practices
AFEB - Armed Forces
Epidemiological Board
AHF - Argentine
hemorrhagic fever
AKD – acetone killed
and dried (typhoid vaccine)
ARD - acute
respiratory disease
CDC - Centers for
Disease Control & Prevention
DoD – U.S.
Department of Defense
DT –
Diphtheria-tetanus toxoids
(pediatric strength)
EEE – eastern equine
encephalitis
FDA - Food & Drug
Administration
GBS - Guillain-Barré
syndrome
HIV - human
immunodeficiency virus
IGIM - immune
globulin intramuscular
JE – Japanese
encephalitis
NAS - National
Academy of Sciences
SKB - SmithKline
Beecham
Td -
tetanus-diphtheria toxoids (adult
strength)
Tdap -
tetanus-diphtheria-acellular
pertussis vaccine
VAERS - Vaccine
Adverse Event Reporting System
VEE – Venezuelan
equine encephalitis
WEE – western equine
encephalitis
WRAIR – Walter Reed
Army Institute of Research
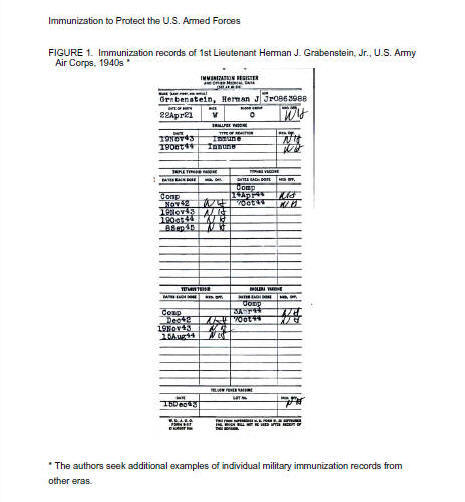
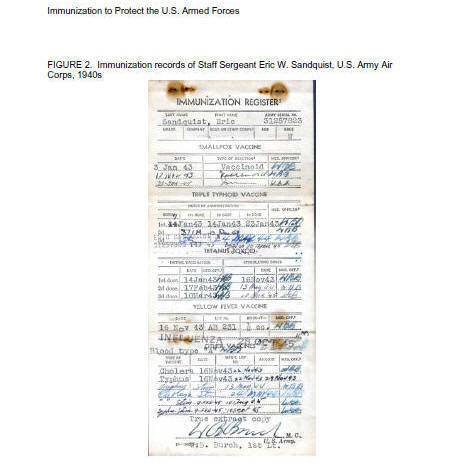
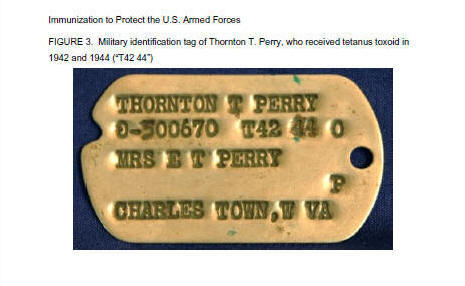
REFERENCES
1.
Takafuji ET, Russell PK.
Military immunizations:
Past, present, and
future prospects. Infect
Dis Clin N
Amer
1990;4:143-58.
2.
Woodward TE. The
public's debt to
military medicine. Mil
Med 1981;146:168-73.
3.
Benenson AS.
Immunization and
military medicine. Rev
Infect Dis 1984;6:1-12.
4.
Parish HJ. A History of
Immunizations. Edinburgh
& London: E&S
Livingstone Ltd, 1965.
5.
Hospenthal DR. History
of U.S. military
contributions to the
understanding,
prevention, and
treatment of
infectious diseases: An
overview. Mil Med
2005;170(4 Suppl):1-2.
6.
Artenstein AW, Opal JM,
Opal SM, Tramont EC,
Peter G, Russell PK.
History of U.S. military
contributions to the
study of vaccines
against infectious
diseases. Mil Med
2005;170(Suppl):3-11.
7.
Grabenstein JD.
ImmunoFacts: Vaccines
and Immunologic Drugs.
Saint Louis: Wolters
Kluwer Health,
2006.
8. U.S.
Department of Defense.
U.S. Army Regulation
40-562; Navy Bureau of
Medicine & Surgery
Instruction 6230.15; Air
Force Joint Instruction
48-110; Coast Guard
Commandant Instruction
M6230.4F.
Immunizations &
Chemoprophylaxis.
Washington, DC, 2005:in
press.
9.
Woodward TE. The Armed
Forces Epidemiological
Board: Its First 50
Years. Falls Church, VA:
Department of the Army,
1990. http://history.amedd.army.mil/booksdocs/itsfirst50yrs/default.htm
10.
Armed Forces
Epidemiological Board.
Vaccines in the
Military: A Department
of Defense-Wide Review
of
Vaccine
Policy and Practice.
Washington, DC:
Department of Defense:
August 1999.
www.ha.osd.mil/afeb/reports/vaccines.pdf
11.
Gillett MC. The Army
Medical Department,
1775-1818. Washington,
DC, U.S. Army Center of
Military
History,
1981:75. http://history.amedd.army.mil/booksdocs/rev/gillett1/frameindex.html
12.
Chase A. Magic Shots.
New York: William
Morrow, 1982.
13.
Plotkin SL, Plotkin SA.
A short history of
vaccination. In: Plotkin
SA, Orenstein WB, ed.
Vaccines, 4th ed.
Philadelphia: Elsevier,
2003:1-12.
14. Long
AP. The Army
immunization program.
In: Coates JB Jr., Hoff
EC, ed.
Medical Department,
U.S.
Army, in
World War II—Preventive
Medicine in World War
II, Volume III: Personal
Health Measures and
Immunization .
Washington, DC: Dept
Army, 1955:271-341.
http://history.amedd.army.mil/booksdocs/wwii/PrsnlHlthMsrs/chapter8.htm
15. Long
AP. Immunizations in the
United States Army. Am J
Public Health
1944;34:27-33.
16.
Bayne-Jones S. The
Evolution of Preventive
Medicine in the United
States Army, 1607-1939.
Washington, DC, Office
of the Surgeon General,
Department of the Army,
1968.
http://history.amedd.army.mil/booksdocs/misc/evprev/frameindex.html
17.
Cowdrey AE. The Medics’
War. Washington, DC,
Center of Military
History, United States
Army, 1987:60
-61,
147-148, 224.
18.
Mullins WS (ed). A
Decade of Progress, The
United States Army
Medical Department,
1959-1969.
Washington, DC, Office
of the Surgeon General,
Department of the Army,
1986:34.
19.
Fenner F, Henderson DA,
Arita I, Jezek Z, Ladnyi
ID. Smallpox and Its
Eradication. Geneva:
World
Health
Organization, 1988.
20.
Rabasa R. George
Washington and
variolation; Edward
Jenner and vaccination.
JAMA 1986;255:1881.
21.
Bollet AJ. The history
of smallpox and its
influence on military
campaigns: Part 1.
Resident Staff Phys
1988;34:135-40.
22.
Sherk HH. Smallpox at
the Morristown
encampment. N J Med
2001;98(11):41-6.
23. Fenn
EA. Pox Americana: The
Great Smallpox Epidemic
of 1775-82. NY: Hill &
Wang, 2001.
24.
Freemon FR.
Administration of the
Medical Department of
the Confederate States
Army,
1861 to 1865. South Med
J 1987;80:630-7.
25.
Bollet AJ. Military
medicine in the
Spanish-American War.
Perspect Biol Med
2005;48:293-300.
26.
Potter LA. Smallpox. In:
Coates JB Jr., Hoff EC,
ed. Medical Department,
United States Army, in
World
War
II—Preventive Medicine
in World War II, Volume
IV: Communicable
Diseases Transmitted
Chiefly
Through
Respiratory & Alimentary
Tracts. Washington, DC:
Department of the Army,
1958:151-63.
27.
Higgins CM.
Horrors of
Vaccination Exposed and
Illustrated.
New York: DeVinne Press,
1920.
28.
Grabenstein JD,
Winkenwerder W Jr. U.S.
military smallpox
vaccination program
experience. JAMA
2003;289:3278-82.
29.
Mendez E Jr. Temporary
discontinuation of
routine smallpox
vaccinations for
military recruits.
Washington, DC:
Department of Defense,
March 19, 1990
(memorandum).
30. Hall
RD. Rabies in Europe:
History and military
impact. Med Bull U.S.
Army Europe
1981;38(Sep):20-4.
Immunization to Protect
the U.S. Armed Forces
28
31.
Bayne-Jones S. Walter
Reed (1851-1902). Mil
Med 1967;132:391-400.
32.
Tigertt WD. The initial
effort to immunize
American soldier
volunteers with typhoid
vaccine. Mil Med
1959;124:342-9.
33. Lim
ML, Murphy GS, Calloway
M, Tribble D. History of
U.S. military
contributions to the
study of diarrheal
diseases. Mil Med
2005;170(4 Suppl):30-8.
34. Reed
W, Vaughan VC,
Shakespeare EO.
Preliminary report on
typhoid fever in
military camps in the
US,
1898.
Army Medical Bulletin
No. 53. Army Medical
Field Service School,
Carlisle Barracks, PA,
Jul
1940.
35.
Vaughn VC. The etiology
and spread of typhoid
fever. JAMA
1902;38:979-88.
36.
Wright AE, Leishman WB.
Remarks on the results
which have been obtained
by the antityphoid
inoculations and on the
methods which have been
employed in the
preparation of the
vaccine. Brit Med J
1900;i:122-9.
37.
Hardy A. “Straight back
to barbarism”:
Antityphoid inoculation
an the Great War, 1914.
Bull Hist Med
2000;74:265-90.
38.
Cantlie N.
History of the Army
Medical Department,
Vol. II. Edinburgh and
London: Churchill
Livingstone,
1974:230, 373.
39.
Russell FF. The control
of typhoid in the Army
by vaccination. NY State
J Med 1910;10:535-48.
40.
Russell FF. Antityphoid
vaccination. Am J Med
Sci 1913;146:803-33.
41. Holt
RL, Hitchens AP. Typhoid
vaccine: The technique
of its preparation at
the Army Medical School.
Public
Health Reports
1937;52:829-44.
42.
Callender GR, Luippold
GF. The effectiveness of
typhoid vaccine prepared
by the U.S. Army. JAMA
1943;
123:319-21.
43.
Vaughan VC, Palmer GT.
Communicable disease in
the United States Army
during the summer and
autumn
of 1918. J Lab Clin Med
1918;3:587-623, 647-86.
44.
Landy M, Gaines S, Seal
JR, Whiteside JE.
Antibody responses of
man to three types of
antityphoid
immunizing agents:
Heat-phenol fluid
vaccine,
acetone-dehydrated
vaccine, and isolated Vi
and O
antigens. Am J Public
Health 1954;44:1572-9.
45.
Simanjuntak CH,
Paleologo FP, Punjabi
NH, Darmowigoto R,
Soeprawoto, Totosudirjo
H, Haryanto P,
Suprijanto E, Witham ND,
Hoffman SL. Oral
immunisation against
typhoid fever in
Indonesia with Ty21a
vaccine.
Lancet 1991;338:1055-9.
46.
Steihm ER. Standard and
special human immune
serum globulins as
therapeutic agents.
Pediatrics
1979;63:301-19.
47. Lévy
FM. A corner of history:
The fiftieth anniversary
of diphtheria and
tetanus immunization.
Prevent
Med
1975;4:226-37.
48. Long
AP. Tetanus toxoid, its
use in the United States
Army. Am J Public Health
1943;33:53-7.
49.
Gottlieb S, McLaughlin
FX, Levine L, Latham WC,
Edsall G. Long-term
immunity to tetanus—A
statistical
evaluation and its
clinical implications.
Am J Public Health
1964;54:961-71.
50.
Jansen DL, Gray GC,
Putnam SD, Lynn F, Meade
BD. Evaluation of
pertussis in U.S. Marine
Corps
trainees. Clin Infect
Dis 1997;25:1099-107.
51.
Vincent JM, Cherry JD,
Nauschuetz WF, Lipton A,
Ono CM, Costello CN,
Sakaguchi LK, Hsue G,
Jackson
LA, Tachdjian R, Cotter
PA, Gornbein JA.
Prolonged afebrile
nonproductive cough
illnesses in
American
soldiers in Korea: A
serological search for
causation. Clin Infect
Dis 2000;30:534-9.
52. Paul
JR. Armed Forces
Epidemiological Board
hepatitis researches: A
review. 1962;127:987-93.
53.
Dooley DP. History of
U.S. military
contributions to the
study of viral
hepatitis. Mil Med
2005;170(4
Suppl):71-6.
54.
Rogers JA. The outbreak
of jaundice in the Army.
JAMA 1942;120:51-3.
55. Cohn
EJ, Oncley JL, Strong
LE, Hughes WL Jr,
Armstrong SH Jr.
Chemical, clinical, and
immunological
studies
on the products of human
plasma fractionation. I.
The characterization of
the protein fractions of
human
plasma. J Clin Invest
1944;23:417-32.
56.
Havens WP Jr. Viral
Hepatitis. In: Havens WP
Jr., ed. Internal
Medicine in World War
II. Vol III:
Infectious Diseases and
General Medicine.
Washington, DC, Office
of the Surgeon General,
Department
of the
Army, 1968:331-84.
http://history.amedd.army.mil/booksdocs/wwii/internalmedicinevolIII/chapter13.htm
57.
Department of Defense.
Prophylactic gamma
globulin for prevention
of endemic hepatitis.
Effects of U.S.
gamma
globulin upon the
incidence of viral
hepatitis and other
infectious diseases in
U.S. Soldiers
abroad.
Arch Intern Med
1971;128:723-38.
58.
Grossman EB, Stewart SG,
Stokes J Jr.
Post-transfusion
hepatitis in battle
casualties and a study
of its
Immunization to Protect
the U.S. Armed Forces
29
prophylaxis by means of
human immune serum
globulin. JAMA
1945;129:991-4.
59.
Stokes J Jr., Neefe JR.
The prevention and
attenuation of
infectious hepatitis by
gamma globulin:
Preliminary Note. JAMA
1945:127:144-5.
60.
Conrad ME. Infectious
hepatitis in military
populations: Problems
encountered with gamma
globulin
prophylaxis. Bull NY
Acad Med 1969;45:167-80.
61.
Lemon SM, Gates N,
Bancroft WH. Prevention
of hepatitis with gamma
globulin. Ann Intern Med
1980;92:868-70.
62.
Conrad ME, Lemon SM.
Prevention of endemic
icteric viral hepatitis
by administration of
immune serum
gamma
globulin. J Infect Dis
1987;156:56-63.
63.
Innis BL, Snitbhan R,
Kunasol P, Laorakpongse
T, Poopatanakool W,
Kozik CA, Suntayakorn S,
Suknuntapong T, Safary
A, Tang DB, Boslego JW.
Protection against
hepatitis A by an
inactivated
vaccine.
JAMA 1994;271:1328-34.
64. Hoke
CH Jr., Binn LN, Egan JE,
DeFraites RF, MacArthy
PO, Innis BL, Eckels KH,
Duboid D, D’Hondt E,
Sjogren
MH, Rice R, Sadoff JC,
Bancroft WH. Hepatitis A
in the U.S. Army:
Epidemiology and vaccine
development. Vaccine
1992;10(Suppl):S75-S79.
65.
Rubertone MV, DeFraites
RF, Krauss MR, Brandt
CA. An outbreak of
hepatitis A during a
military field
training
exercise. Mil Med
1993;158:37-41.
66.
Centers for Disease
Control & Prevention.
Health Information for
International Travel,
2005-2006.
Atlanta,
U.S. Department of
Health and Human
Services, 2005.
67.
Krugman S. The
Willowbrook hepatitis
studies revisited:
Ethical aspects. Rev
Infect Dis
1986:8:157-62.
68.
Sawyer WA, Meyer KF,
Eaton MD, Bauer JH,
Putnam P, Schwentker FF.
Jaundice in Army
personnel in
the
western region of the
United States and its
relation to vaccination
against yellow fever:
Part I. Am J
Hyg
1944;39:337-430.
69.
Sawyer WA, Meyer KF,
Eaton MD, Bauer JH,
Putnam P, Schwentker FF.
Jaundice in Army
personnel in
the
western region of the
United States and its
relation to vaccination
against yellow fever:
Parts II, III
and IV.
Am J Hyg 1944;40:35-107.
70.
Bancroft HW, Kelley PW,
Takafuji ET. The
military and hepatitis
B. Vaccine
1990;8(Suppl):S33-S36.
71.
Lemon SM, Miller RN,
Pang LW, Prier RE,
Bernard KW. Failure to
achieve predicted
antibody responses
with
intradermal and
intramuscular human
diploid cell rabies
vaccine. Lancet
1984;i:1098-100.
72.
Ronish RH, Diniega BM,
Kelley PW, Sjogren MH,
Arday DR, Aronson NE,
Hoke CH, Petruccelli BP.
Immunogenicity achieved
by the intradermal
hepatitis B vaccination
programme for U.S. Army
soldiers in
Korea.
Vaccine 1991;9:364-8.
73.
Taylor DN, Sanchez JL,
Castro JM, Lebron C,
Parrado CM, Johnson DE,
Tacket CO, Losonsky GA,
Wasserman SS, Levine MM,
Cryz SJ. Expanded safety
and immunogenicity of a
bivalent, oral,
attenuated cholera
vaccine, CVD 103-HgR
plus CVD 111, in United
States military
personnel stationed
in
Panama. Infect Immun
1999;67:2030-4.
74.
Taylor DN, Cardenas V,
Sanchez JL, Begue RE,
Gilman R, Bautista C,
Perez J, Puga R,
Gaillour A,
Meza R,
Echeverria P, Sadoff J.
Two-year study of the
protective efficacy of
the oral whole cell plus
recombinant B subunit
cholera vaccine in Peru.
J Infect Dis
2000;181:1667-73.
75.
Blood CG, Jolly R.
Comparisons of disease
and nonbattle injury
incidence across various
military
operations. Mil Med
1995;160:258-63.
76.
Vaughan VC. An explosive
epidemic of influenzal
disease at Fort
Oglethorpe. J Lab Clin
Med
1918;3:560-4
(editorial).
77.
Soper GA. The influenza
pneumonia pandemic in
the American Army camps
during September and
October,
1918. Science
1918;68:451-6.
78.
Bollet A. President
Wilson and the
blitzkatarrh. Resid
Staff Phys
1988;34:183-7, 191.
79.
Kolata G.
Flu: The Story of the
Great Influenza Pandemic
of 1918 and the Search
for the Virus That
Caused
It.
New York: Farrar Straus
Giroux, 1999.
80.
Leary T. The use of
influenza vaccine in the
present epidemic. Am J
Public Health
1918;8:754-5, 768.
81.
Anonymous. Vaccines
against influenza.
Public Health Reports
1918;33:1866.
82.
Anonymous. Value of
vaccination against
influenza. JAMA
1918;71:1583
(editorial).
83.
Siler JF. The U.S. Army
Medical Department in
the World War. Vol. IX.
Communicable and Other
Diseases. Washington,
DC: United States Army,
1928:66-70.
http://history.amedd.army.mil/booksdocs/wwi/communicablediseases/default.htm
84.
Francis T Jr. The
development of the 1943
vaccination study of the
Commission on Influenza.
Am J Hyg
1945;42:1-8.
85. Salk
JE, Menke WJ Jr, Francis
T Jr. A clinical,
epidemiological, and
immunological evaluation
of
Immunization to Protect
the U.S. Armed Forces
30
vaccination against
epidemic influenza. Am J
Hyg 1945;42:57-93.
86.
Takafuji ET, Johnson DE,
Segal HE. The swine
antigen in previous
influenza vaccines. N
Engl J Med
1976;295:1018 (letter).
87.
Meiklejohn G. Viral
respiratory disease at
Lowry Air Force Base in
Denver, 1952-1982. J
Infect Dis
1983;148:775-84.
88.
Hilleman MR. Personal
historical chronicle of
six decades of basic and
applied research in
virology,
immunology, and
vaccinology. Immunol Rev
1999;170:7-27.
89.
Davenport FM. Seventeen
years’ experience with
mineral oil adjuvant
influenza virus
vaccines. Ann
Allergy
1968;26:288-92.
90.
Beebe GW, Simon AH,
Vivona S. Long-term
mortality follow-up of
Army recruits who
received adjuvant
influenza virus vaccine
in 1951—1953. Am J
Epidemiol
1972;95;337-46.
91.
Gaydos JC, Hodder RA,
Top FH Jr., Soden VJ,
Allen RG, Bartley JD,
Zabkar JH, Nowosiwsky T,
Russell
PK.
Swine influenza A at
Fort Dix, New Jersey
(January-February 1976).
I. Case finding and
clinical
study of
cases. J Infect Dis
1977;136(Suppl):S356-S362.
92.
Gaydos JC, Hodder RA,
Top FH Jr., Allen RG,
Soden VJ, Nowosiwsky T,
Russell PK. Swine
influenza A
at Fort
Dix, New Jersey
(January-February 1976).
II. Transmission and
morbidity in units with
cases. J
Infect
Dis
1977;136(Suppl):S363-S368.
93.
Hodder RA, Gaydos JC,
Allen RG, Top FH Jr.,
Nowosiwsky T, Russell
PK. Swine influenza A at
Fort
Dix, New
Jersey (January-February
1976). III. Extent of
spread and duration of
the outbreak. J Infect
Dis
1977;136(Suppl):S369-S375.
94. Top
FH Jr., Russell PK.
Swine influenza A at
Fort Dix, New Jersey
(January-February 1976).
IV.
Summary
and speculation. J
Infect Dis
1977;136:S376-S380.
95.
Neustadt R, Fineberg HV.
The Epidemic That
Never Was: Policy Making
in the Swine Flu Scare.
New
York:
Vintage Books, 1983.
96. Wade
N. 1976 swine flu
campaign faulted yet
principals would do it
again. Science
1978;202:849-50.
97.
Schonberger LB, Bregman
DJ, Sullivan-Bolyai JZ,
Keenlyside RA, Ziegler
DW, Retailliau HF,
Eddins DL,
Bryan
JA. Guillain-Barre
syndrome following
vaccination in the
National Influenza
Immunization
Program,
United States,
1976--1977. Am J
Epidemiol
1979;110:105-23.
98.
Langmuir AD, Bregman DJ,
Kurland LT, Nathanson N,
Victor M. An
epidemiologic and
clinical evaluation
of
Guillain-Barre syndrome
reported in association
with the administration
of swine influenza
vaccines.
Am J
Epidemiol
1984;119:841-79.
99.
Breman JG, Hayner NS.
Guillain-Barre syndrome
and its relationship to
swine influenza
vaccination in
Michigan, 1976-1977. Am
J Epidemiol
1984;119:880-9.
100.
Safranek TJ, Lawrence DN,
Kurland LT, Culver DH,
Wiederholt WC, Hayner
NS, Osterholm MT,
O'Brien
P, Hughes JM.
Reassessment of the
association between
Guillain-Barre syndrome
and receipt
of swine
influenza vaccine in
1976-1977: Results of a
two-state study. Am J
Epidemiol
1991;133:940-51.
101.
Johnson DE.
Guillain-Barre syndrome
in the U.S. Army. Arch
Neurol 1982;39:21-4.
102.
Earhart KC, Beadle C,
Miller LK, Pruss MW,
Gray GC, Ledbetter EK,
Wallace MR. Outbreak of
influenza in highly
vaccinated crew of U.S.
Navy ship.
Emerg Infect Dis
2001;7:463-5.
103.
Canas LC, Lohman K,
Pavlin JA, Endy T, Singh
DL, Pandey P, Shrestha
MP, Scott RMc, Russell
KL,
Watts D,
Hajdamowicz M, Soriano
I, Douce RW, Neville J,
Gaydos JC. The
Department of Defense
Laboratory-Based Global
Influenza Surveillance
System. Mil Med
2000;165:52-6.
104.
Goldschneider I,
Gotschlich EC,
Artenstein MS. Human
immunity to the
meningococcus. I. The
role of
human
immunity. J Exp Med
1969; 129:1307-26.
105.
Goldschneider I,
Gotschlich EC,
Artenstein MS. Human
immunity to the
meningococcus. II. The
development of natural
immunity. J Exp Med
1969; 129:1327-48.
106.
Gotschlich EC,
Goldschneider I,
Artenstein MS. Human
immunity to the
meningococcus. IV.
Immunogenicity of group
A and group C
meningococcus
polysaccharides in human
volunteers. J Exp
Med
1969;129:1367-84.
107.
Brundage JF, Ryan MA,
Feighner BH, Erdtmann
FJ. Meningococcal
disease among United
States
military
service members in
relation to routine uses
of vaccines with
different
serogroup-specific
components, 1964-1998.
Clin Infect Dis
2002;35:1376-81.
108.
Advisory Committee on
Immunization Practices.
Prevention & control of
meningococcal disease;
Meningococcal disease
and college students.
MMWR 2000;49(RR-7):1-20.
109.
Hilleman MR, Werner JH,
Adair CV, Dreisbach AR.
Outbreak of acute
respiratory illness
caused by RI-
67 and
influenza A viruses,
Fort Leonard Wood,
1952-1953. Am J Hyg
1955;61:163-73.
110.
Hilleman MR, Stallones
RA, Gauld RL, Warfield
MS. Anderson SA.
Vaccination against
acute
Immunization to Protect
the U.S. Armed Forces
31
respiratory illness of
adenovirus (RI-APC-ARD)
etiology. Am J Public
Health 1957;47:841-7.
111.
Buescher EL. Respiratory
disease and the
adenoviruses. Med Clin
North Am 1967;51:769-79.
112. Top
FH Jr, Grossman RA,
Bartelloni PI, Segal HE,
Dudding BA, Russell PK,
Buescher EL.
Immunization with live
types 7 and 4 vaccines.
I. Safety, infectivity,
antigenicity, and
potency of
adenovirus type 7
vaccine in humans, J
Infect Dis
1971;124:148-54.
113.
Dudding BA, Top FH Jr.,
Scott RM, Russell PK,
Buescher EL. An analysis
of hospitalizations for
acute
respiratory disease in
recruits immunized with
adenovirus type 4 and
type 7 vaccines. Am J
Epidemiol
1972;95:140-7.
114.
Dudding BA, Top FH Jr,
Winter PE, Buescher EL,
Lamson TH, Leibovitz A.
Acute respiratory
disease in
military
trainees. Am J Epidemiol
1973;97:187-98.
115.
Colis PB, Dudding BA,
Winter PE, Russell PK,
Buescher EL. Adenovirus
vaccines in military
recruit
populations: A
cost-benefit analysis. J
Infect Dis
1973:128:745-52.
116. Top
FH Jr. Control of
adenovirus acute
respiratory disease in
U.S. Army trainees. Yale
J Biol Med
1975;48:185-95.
117.
Takafuji ET, Gaydos JC,
Allen RG, Top FH Jr.
Simultaneous
administration of live,
enteric-coated
adenovirus types 4, 7,
and 21 vaccines: Safety
and immunogenicity. J
Infect Dis
1979;140:48-53.
118.
Gaydos CA, Gaydos JC.
Adenovirus vaccines in
the U.S. Military. Mil
Med 1995; 160:300-4.
119.
Culver JO, Lennette EH,
Flintjer JD. Adenovirus
vaccine: A field
evaluation of protective
efficacy against
respiratory disease. Am
J Hyg 1959;69:120-6.
120.
Brown F, Lewis AM, eds.
Simian virus 40 (SV40):
A possible human
polyomavirus, Symposium
proceedings. Dev Biol
Stand 1998;94:1-406.
121.
Strickler HD, Rosenberg
PS, Devesa SS, Hertel J,
Fraumeni JF, Goedert JJ.
Contamination of
poliovirus vaccines with
simian virus 40
(1955-1963) and
subsequent cancer rates.
JAMA 1998;279:292-
5.
122.
Institute of Medicine.
Immunization Safety
Review: Simian Virus-40
Contamination of Polio
Vaccine and
Cancer.
Washington, DC: National
Academy of Sciences, Oct
2002.
www.nap.edu/catalog/10534.html
123.
Rollison DE, Page WF,
Crawford H, Gridley G,
Wacholder S, Martin J,
Miller R, Engels EA.
Casecontrol
study of
cancer among U.S. Army
veterans exposed to
simian virus
40-contaminated
adenovirus
vaccine.
Am J Epidemiol
2004;160:317-24.
124.
Gooch WM III, Mogabgab
WJ. Simultaneous oral
administration of live
adenovirus types 4 and 7
vaccines: Protection and
lack of emergence of
other types. Arch
Environ Health
1972;25:388-94.
125.
Barraza EM, Ludwig SL,
Gaydos JC, Brundage JF.
Reemergence of
adenovirus type 4 acute
respiratory disease in
military trainees:
Report of an outbreak
during a lapse in
vaccination. J Infect
Dis
1999;179:1531-3.
126.
Gray GC, Goswami PR,
Malasig MD, Hawksworth
AW, Trump DH, Ryan MA,
Schnurr DP. Adult
adenovirus infections:
Loss of orphaned
vaccines precipitates
military respiratory
disease epidemics.
Clin
Infect Dis
2000;31:663-70.
127.
Centers for Disease
Control & Prevention.
Two fatal cases of
adenovirus-related
illness in previously
healthy
young adults--Illinois,
2000. MMWR
2001;50:553-5.
128.
Ryan MA, Gray GC, Smith
B, McKeehan JA,
Hawksworth AW, Malasig
MD. Large epidemic of
respiratory illness due
to adenovirus types 7
and 3 in healthy young
adults. Clin Infect Dis
2002;34:577-
82.
129.
Hyer RN, Howell MR, Ryan
MA, Gaydos JC.
Cost-effectiveness
analysis of reacquiring
and using
adenovirus types 4 and 7
vaccines in naval
recruits. Am J Trop Med
Hyg 2000;62:613-8.
130.
Lemon SM, Thaul S,
Fisseha S, O’Moonaigh
HC, ed. Protecting Our
Forces: Improving
Vaccine
Acquisition and
Availability in the U.S.
Military. Washington,
DC: National Academy
Press, 2002.
131.
Vaughan VC. Measles and
pneumonia in our camps.
J Lab Clin Med
1918;3:248-57
(editorial).
132.
Byerly CR. Fever of War:
The Influenza Epidemic
in the U.S. Army during
World War I. New York:
New
York
University Press, 2005.
133.
Ottolini MG, Burnett MW.
History of U.S. military
contributions to the
study of respiratory
infections. Mil
Med
2005;170(4 Suppl):66-70.
134.
Crozier D. The military
significance of
infectious disease. Mil
Med 1962;127:392-6.
135.
Parkman PD, Buescher EL,
Artenstein MS. Recovery
of rubella virus from
Army recruits. Proc Soc
Exp
Biol Med
1962;111:225-30.
136.
Katz SL, Enders JF,
Holloway A. The
development and
evaluation of an
attenuated measles virus
vaccine.
Am J Public Health
1962;52:5-10.
137.
Centers for Disease
Control. Measles—U.S.
military. MMWR
1981;30:314-6.
Immunization to Protect
the U.S. Armed Forces
32
138.
Craig SC, Broughton G
2nd, Bean J, McKee KT
Jr. Rubella outbreak,
Fort Bragg, North
Carolina, 1995:
A clash
of two preventive
strategies. Mil Med
1999;164:616-8.
139.
Arday DR, Kanjarpane DD,
Kelley PW. Mumps in the
U.S. Army 1980-86:
Should recruits be
immunized? Am J Public
Health 1989;79:471-4.
140.
Blouse LE, Lathrop GD,
Dupuy HJ, Ball RJ.
Rubella screening and
vaccination program for
U.S. Air
Force
trainees: An analysis of
findings. Am J Public
Health 1982;72:280-3.
141.
Kelley PW, Petruccelli
BP, Stehr-Green P,
Erickson RL, Mason CJ.
The susceptibility of
young adult
Americans to
vaccine-preventable
infections. A national
serosurvey of U.S. Army
recruits. JAMA
1991;266:2724-9.
142.
Clardy WF.
Susceptibility in USAF
recruits to
vaccine-preventable
diseases. Vaccine
1993;11:573-5.
143.
Struewing JP, Hyams KC,
Tueller JE, Gray GC. The
risk of measles, mumps,
and varicella among
young
adults: A serosurvey of
U.S. Navy and Marine
Corps recruits. Am J
Public Health
1993;83:1717-
20.
144.
Smoak BL, Novakoski WL,
Mason CJ, Erickson RL.
Evidence for a recent
decrease in measles
susceptibility among
young American adults. J
Infect Dis
1994;170:216-9.
145.
Burnham BR, Wells TS,
Riddle JR. A
cost-benefit analysis of
a routine varicella
vaccination program
for
United States Air Force
Academy cadets. Mil Med
1998;163:631-4.
146.
Howell MR, Lee T, Gaydos
CA, Nang RN. The
cost-effectiveness of
varicella screening and
vaccination in U.S. Army
recruits. Mil Med
2000;165:309-15.
147.
McGuinness AC.
Diphtheria. In: Coates
JB Jr., Hoff EC, ed.
Medical Department,
United States Army,
in World
War II—Preventive
Medicine in World War
II, Volume IV:
Communicable Diseases
Transmitted
Chiefly
Through Respiratory &
Alimentary Tracts.
Washington, DC:
Department of the Army,
1958:167-
89.
148.
Liebow AA, Bumstead JH.
Cutaneous and other
aspects of diphtheria.
In: Havens WP Jr., ed.
Internal
Medicine
in World War II. Vol II:
Infectious Diseases.
Washington, DC, Office
of the Surgeon General,
Department of the Army,
1963:275-308.
http://history.amedd.army.mil/booksdocs/wwii/infectiousdisvolii/chapter10.htm
149.
Edsall G. Immunization
of adults against
diphtheria and tetanus.
Am J Public Health
1952;42:393-400.
150.
Edsall G, Altman JS,
Gaspar AJ. Combined
tetanus-diphtheria
immunization of adults:
Use of small
doses of
diphtheria toxoid. Am J
Public Health
1954;44:1537-45.
151.
Pierce JR. "In the
interest of humanity and
the cause of science":
The yellow fever
volunteers. Mil Med
2003;168:857-63.
152.
Endy TP, Thomas SJ,
Lawler JV. History of
U.S. military
contributions to the
study of viral
hemorrhagic
fevers.
Mil Med 2005;170(4 Suppl):77-91.
153.
Reed W. Carroll J,
Agramonte A. The
etiology of yellow
fever: An additional
note. JAMA 1901;36:431-
40.
154.
Sternberg GM. The
transmission of yellow
fever by mosquitoes.
Popular Sci Monthly
1901;59:225-41.
155.
Reed W. Recent
researches concerning
the etiology,
propagation, and
prevention of yellow
fever, by
the U.S.
Army Commission. J Hyg
1902;2:101-19.
156.
Reed W. Carroll J,
Agramonte A, Lazear JW.
The etiology of yellow
fever: A preliminary
note. Phila Med
J
1900;6:790-6; Mil Med
2001;166(Suppl 1):29-36.
157.
Chaves-Carballo E.
Carlos Finlay and yellow
fever: Triumph over
adversity. Mil Med
2005;170:881-5.
158.
Blanck RR. The history
of immunization in the
U.S. Armed Forces. Minn
Med 2002;85(2):14-7.
159.
Seeff LB, Beebe GW,
Hoofnagle JH, Norman JE,
Buskell-Bales Z,
Waggoner JG, Kaplowitz
N, Koff RS,
Petrini
JL Jr, Schiff ER, Shorey
J, Stanley MM. A
serologic follow-up of
the 1942 epidemic of
postvaccination
hepatitis in the United
States Army. N Engl J
Med 1987;316:965-70.
160.
Norman JE, Beebe GW,
Hoofnagle JH, Seeff LB.
Mortality follow-up of
the 1942 epidemic of
hepatitis B
in the
U.S. Army. Hepatology
1993;18:790-7.
161.
Waters TD, Anderson PS
Jr., Beebe GW, Miller
RW. Yellow fever
vaccination, avian
leucosis virus, and
cancer
risk in man. Science
1972;177:76-7.
162.
Centers for Disease
Control & Prevention.
Adverse events
associated with
17D-derived yellow fever
vaccination--United
States, 2001-2002. Morb
Mortal Weekly Report
MMWR 2002;51:989-93.
163.
Sabin AB. Epidemic
encephalitis in military
personnel: Isolation of
Japanese B virus on
Okinawa in
1945,
serologic diagnosis,
clinical manifestations,
epidemiological aspects
and use of mouse brain
vaccine.
JAMA 1947;133:281-93.
164.
Tigertt WD, Hammon WMcD,
Berge TO, Blender JX,
Burns KF, Casals J,
Cousins RF, Keegan HL,
Maclaren
JP, Meiklejohn G, Reeves
WC, Rees DM, Sather G.
Japanese B encephalitis:
A complete
Immunization to Protect
the U.S. Armed Forces
33
review
of experience on Okinawa
1945-1949. Am J Trop Med
1950;30:689-721.
165.
Hoke CH, Nisalak A,
Sangawhipa N, Jatanasen
S, Laorakapongse T,
Innis BL, Kotchasesee S,
Gingrich
JB, Latendresse J, Fukai
K, Burke DS. Protection
against Japanese
encephalitis by
inactivated
vaccines. N Engl J Med
1988;319:608-14.
166.
Vaughn DW, Hoke CH Jr.
The epidemiology of
Japanese encephalitis:
Prospects for
prevention.
Epidemiol Rev
1992;14:197-221.
167.
DeFraites RF, Gambel JM,
Hoke CH Jr, Sanchez JL,
Withers BG, Karabatsos
N, Shope RE, TIrrell S,
Yoshida
I, Takagi M, Meschievitz
CK, Tsai TF. Japanese
encephalitis vaccine
(inactivated, Biken) in
U.S.
soldiers: Immunogenicity
and safety of vaccine
administered in two
dosing regimens. Am J
Trop Med
Hyg
1999;61:288-93.
168.
Hoke CH Jr. History of
U.S. military
contributions to the
study of viral
encephalitis. Mil Med
2005;170(4
Suppl):92-105.
169.
Advisory Committee on
Immunization Practices.
Human rabies
prevention--United
States, 1999.
MMWR
1999;48(RR-1):1-21.
Erratum 1999;48:16.
www.cdc.gov/mmwr/PDF/rr/rr4801.pdf
170.
Halsell JS, Riddle JR,
Atwood JE, Gardner P,
Shope R, Poland GA, Gray
GC, Ostroff S, Eckart
RE,
Gibson
RL, Grabenstein JD,
Arness MK, Tornberg DN,
Department of Defense
Smallpox Vaccination
Clinical
Evaluation Team.
Myopericarditis
following smallpox
vaccination among
vaccinia-naïve U.S.
military
personnel. JAMA
2003;289:3283-89.
171.
Arness MK, Eckart RE,
Love SS, Atwood JE,
Wells TS, Engler RJ,
Collins LC, Ludwig SL,
Riddle JR,
Grabenstein JD, Tornberg
DN. Myopericarditis
following smallpox
vaccination. Am J
Epidemiol
2004;160:642-51.
172.
Eckart RE, Love SS,
Atwood JE, Arness MK,
Cassimatis DC, Campbell
CL, Boyd SY, Murphy JG,
Swerdlow
DL, Collins LC, Riddle
JR, Tornberg DN,
Grabenstein JD, Engler
RJM, DoD Smallpox
Vaccination Clinical
Evaluation Team.
Incidence and follow-up
of inflammatory cardiac
complications
following smallpox
vaccination. J Am Coll
Cardiol 2004;44:201-5.
173.
Inglesby TV, O'Toole T,
Henderson DA, Bartlett
JG, Ascher MS, Eitzen E,
Friedlander AM,
Gerberding
J, Hauer
J, Hughes J, McDade J,
Osterholm MT, Parker G,
Perl TM, Russell PK,
Tonat K, Working
Group on
Civilian Biodefense.
Anthrax as a biological
weapon, 2002: Updated
recommendations for
management. JAMA
2002;287:2236-52.
jama.ama-assn.org/cgi/reprint/287/17/2236.pdf
174.
Jernigan DB, Raghunathan
PL, Bell BP, Brechner R,
Bresnitz EA, Butler JC,
Cetron M, Cohen M, Doyle
T,
Fischer M, Greene C,
Griffith KS, Guarner J,
Hadler JL, Hayslett JA,
Meyer R, Petersen LR,
Phillips
M,
Pinner R, Popovic T,
Quinn CP, Reefhuis J,
Reissman D, Rosenstein
N, Schuchat A, Shieh WJ,
Siegal
L, Swerdlow DL, Tenover
FC, Traeger M, Ward JW,
Weisfuse I, Wiersma S,
Yeskey K, Zaki S,
Ashford
DA, Perkins BA, Ostroff
S, Hughes J, Fleming D,
Koplan JP, Gerberding
JL; National Anthrax
Epidemiologic
Investigation Team.
Investigation of
bioterrorism-related
anthrax, United States,
2001:
Epidemiologic findings.
Emerg Infect Dis
2002;8:1019-28.
www.cdc.gov/ncidod/EID/vol8no10/pdf/02-
0353.pdf
175.
Wright GG, Green TW,
Kanode RG. Studies on
immunity in anthrax. V.
Immunizing activity of
alumprecipitated
protective antigen. J
Immunol 1954;73:387-91.
176.
Wright GG, Puziss M,
Neely WB. Studies on
immunity in anthrax. IX.
Effect of variations in
cultural
conditions on
elaboration of
protective antigen by
strains of
Bacillus anthracis.
Journal of
Bacteriology
1962;83:515-22.
177.
Puziss M, Wright GG.
Studies on immunity in
anthrax. X. Gel-adsorbed
protective antigen for
immunization of man. J
Bacteriol 1963;85:230-6.
178.
Covert NM. Cutting Edge:
A History of Fort
Detrick, Maryland, 3 rd
ed. Fort Detrick: U.S.
Army Garrison,
1997.
179.
Joellenbeck LM,
Zwanziger L, Durch JS,
Strom BL, editors.
The Anthrax Vaccine:
Is it Safe? Does it
Work?
Washington, DC: National
Academy Press, April
2002,
www.nap.edu/catalog/10310.html.
180.
Christopher GW, Agan MB,
Cieslak TJ, Olson PE.
History of U.S. military
contributions to the
study of
bacterial zoonoses. Mil
Med 2005;170(4
Suppl):39-48.
181.
Brachman PS, Gold H,
Plotkin SA, Fekety FK,
Werrin M, Ingram NR.
Field evaluation of
human anthrax
vaccine.
American Journal of
Public Health
1962;52:632-45.
www.anthrax.mil/media/pdf/field_eval.pdf
182.
Advisory Committee on
Immunization Practices.
Use of anthrax vaccine
in the United States.
MMWR
2000;49(RR-15):1-20.
www.cdc.gov/mmwr/PDF/rr/rr4915.pdf
183.
Food & Drug
Administration.
Biological products;
Bacterial vaccines and
toxoids; Implementation
of
efficacy
review; Anthrax vaccine
adsorbed; Final order.
Fed Reg
2005;70:75180-98.
www.fda.gov/cber/rules/bvactoxanth.pdf
Immunization to Protect
the U.S. Armed Forces
34
184.
Berezuk GP, McCarty GE.
Investigational drugs
and vaccines fielded in
support of Operation
Desert
Storm.
Mil Med 1992;157:404-6.
185.
McKee KT Jr, Berezuk GP,
Balady MA. Medicine and
war. N Engl J Med
1992;327:1096-7.
186.
Nightingale SL. Medicine
and war. N Engl J Med.
1992;327:1097-8.
187.
Schuchardt EJ.
Distinguishing between
research and medical
practice during
Operation Desert Storm.
Food
Drug Law J
1994;49:271-89.
188.
Department of Defense.
Vaccine use during the
Gulf War. Dec 7, 2000.
www.gulflink.osd.mil/va/.
Accessed
October 10, 2005.
189.
Pittman PR, Gibbs PH,
Cannon TL, Friedlander
AM. Anthrax vaccine:
Short-term safety
experience in
humans.
Vaccine
2001;20:972-8.
www.vaccines.mil/documents/library/Pittman2002Vaccine972.pdf
190.
Pittman PR, Mangiafico
JA, Rossi CA, Cannon TL,
Gibbs PH, Parker GW,
Friedlander AM. Anthrax
vaccine:
Increasing intervals
between the first two
doses enhances antibody
response in humans.
Vaccine
2001;18:213-6.
www.vaccines.mil/documents/library/Vaccine19(2-3)213-6.pdf
191.
Gunzenhauser JD, Cook
JE, Parker ME. Acute
side effects of anthrax
vaccine in ROTC cadets
participating in
advanced camp, Fort
Lewis, 2000.
Medical Surveillance
Monthly Report (MSMR)
2001;7(5):9-11.
amsa.army.mil/1MSMR/2001/v07_n05.pdf
192.
Pittman PR, Kim-Ahn G,
Pifat DY, Coonan K,
Gibbs P, Little S,
Pace-Templeton JG, Myers
R, Parker
GW,
Friedlander AM. Anthrax
vaccine: Safety and
immunogenicity of a
dose-reduction, route
comparison study in
humans.
Vaccine
2002;20:1412-20.
www.vaccines.mil/documents/library/Immunogenicity.pdf
193.
Rehme PA, Williams R,
Grabenstein JD.
Ambulatory medical
visits among anthrax
vaccinated and
unvaccinated personnel
after return from
southwest Asia.
Military Medicine
2002;167:205-10.
www.anthrax.mil/documents/library/SWasia.pdf
194.
Pittman PR, Hack D,
Mangiafico J, Gibbs P,
McKee KT Jr., Eitzen EM,
Friedlander AM, Sjogren
MH.
Antibody
response to a delayed
booster dose of anthrax
vaccine and botulinum
toxoid.
Vaccine
2002;20:
2107-15.
www.vaccines.mil/documents/library/Antibody_resp.pdf
195.
Wasserman GM,
Grabenstein JD, Pittman
PR, Rubertone MV, Gibbs
PP, Wang LZ, Golder LG.
Analysis
of adverse events after
anthrax vaccination in
U.S. Army medical
personnel.
Journal of
Occupational &
Environmental Medicine
2003;45:222-33.
www.anthrax.mil/documents/library/AnthraxVaccineEvaluation.pdf
196.
Hoffman K, Costello C,
Menich M, Grabenstein
JD, Engler RJM. Using a
structured medical note
for
determining the safety
profile of anthrax
vaccine for U.S.
Soldiers in Korea.
Vaccine
2003;21:4399-409.
www.vaccines.mil/documents/library/Hoffman2003Vaccine4399.pdf
197.
Hunter D, Zoutman D,
Whitehead J, Hutchings
J. Health effects of
anthrax vaccination in
the Canadian
Forces.
Military Medicine
2004;169:833-838.
www.anthrax.mil/documents/library/anthraxvaccinestudy.pdf
198.
Lange JL, Lesikar SE,
Brundage JF, Rubertone
MV. Comprehensive
systematic surveillance
for
adverse
effects of anthrax
vaccine adsorbed, U.S.
Armed Forces, 1998-2000.
Vaccine
2003;21:1620-28.
www.anthrax.mil/documents/library/science.pdf
199.
Sulsky SI, Grabenstein
JD, Delbos RG.
Disability among U.S.
Army personnel
vaccinated against
anthrax.
J Occup Environ Med
2004;46:1065-75.
www.anthrax.mil/documents/library/Anthrax2004.pdf
200.
Wiesen AR, Littell CT.
Relationship between
prepregnancy anthrax
vaccination and
pregnancy and birth
outcomes
among U.S. Army women.
JAMA
2002;287:1556-60.
jama.amaassn.
org/cgi/reprint/287/12/1556.pdf
201.
Catherino WH, Levi A,
Kao T-C, Leondires MP,
McKeeby J, Segars JH.
The anthrax vaccine does
not
affect
semen parameters, embryo
quality, or pregnancy
outcome in couples with
a vaccinated male
military
service member.
Fertility Sterility
2005;83:480-3.
www.vaccines.mil/documents/library/Catherino_Anthrax_Vaccine_2005.pdf
202.
Centers for Disease
Control and Prevention.
Status of U.S.
Department of Defense
preliminary
evaluation of the
association of anthrax
vaccination and
congenital anomalies.
MMWR 2002;51:127.
www.cdc.gov/mmwr/PDF/wk/mm5106.pdf
203.
Sever JL, Brenner AI,
Gale AD, Lyle JM,
Moulton LH, West DJ.
Safety of anthrax
vaccine: A review by
the
Anthrax Vaccine Expert
Committee (AVEC) of
adverse events reported
to the Vaccine Adverse
Event
Reporting System
(VAERS).
Pharmacoepid Drug Saf
2002;11:189-202.
www.anthrax.mil/media/pdf/AVEC_ms.pdf
204.
Sever JL, Brenner AI,
Gale AD, Lyle JM,
Moulton LH, Ward BJ,
West DJ. Safety of
anthrax vaccine: An
expanded
review and evaluation of
adverse events reported
to the Vaccine Adverse
Event Reporting
Immunization to Protect
the U.S. Armed Forces
35
System (VAERS).
Pharmacoepid Drug Saf
2004;13:825-40.
www.anthrax.mil/media/pdf/SeverArticle.pdf
205.
Pittman PR, Coonan KM,
Gibbs PH, Scott HM,
Cannon TL, McKee KT Jr.
Long-term health effects
of
repeated
exposure to multiple
vaccines.
Vaccine
2004;23:525-36.
www.vaccines.mil/documents/library/Longtermhealtheffects.pdf
206.
Matyas GR, Rao M,
Pittman PR, Burge R,
Robbins IE, Wassef NM,
Thivierge B, Alving CR.
Detection of
antibodies to squalene:
III. Naturally occurring
antibodies to squalene
in humans and mice. J
Immunol
Methods.
2004;286:47-67.
207.
Meyer KF, Cavanaugh DC,
Bartelloni PJ, Marshall
JD Jr. Plague
immunization. I. Past
and present
trends.
J Infect Dis
1974;129(Suppl):S13-S18.
208.
Marshall JD Jr,
Bartelloni PJ, Cavanaugh
DC, Kadull PJ, Meyer KF.
Plague immunization. II.
Relation of
adverse
clinical reactions to
multiple immunizations
with killed vaccine. J
Infect Dis
1974;129(Suppl):S19-S25.
209.
Cavanaugh DC, Cadigan FC
Jr., Williams JE,
Marshall JD Jr. Plague.
In: Ognibene AJ, Barrett
O Jr.,
ed.
Internal Medicine in
Vietnam. Volume II.
General Medicine and
Infectious Diseases.
Washington,
DC: U.S.
Army, 1982:167-97.
210.
Titball RW, Williamson
ED. Vaccination against
bubonic and pneumonic
plague. Vaccine
2001;19:4175-
84.
211.
Titball RW, Williamson
ED. Yersinia pestis
(plague) vaccines.
Expert Opinion Biol Ther
2004;4:965-73.
212.
Advisory Committee on
Immunization Practices.
Prevention of plague.
MMWR
1996;45(RR-14):1-16.
ftp://ftp.cdc.gov/pub/Publications/mmwr/rr/rr4514.pdf
213.
Inglesby TV, Dennis DT,
Henderson DA, Bartlett
JG, Ascher MS, Eitzen E,
Fine AD, Friedlander AM,
Hauer J,
Koerner JF, Layton M,
McDade J, Osterholm MT,
O'Toole T, Parker G,
Perl TM, Russell PK,
Schoch-Spana M, Tonat K,
Working Group on
Civilian Biodefense.
Plague as a biological
weapon:
Medical
and public health
management. JAMA
2000;283:2281-90.
jama.amaassn.
org/cgi/reprint/283/17/2281.pdf
214.
Russell P, Eley SM,
Hibbs SE, Manchee RJ,
Stagg AJ, Titball RW. A
comparison of plague
vaccine,
USP, and
EV76 vaccine induced
protection against
Yersinia pestis in a
murine model. Vaccine
1995;13:1551-6.
215.
Heath DG, Anderson GW
Jr., Mauro JM, Welkos
SL, Andrews GP,
Adamovicz J, Friedlander
AM.
Protection against
experimental bubonic and
pneumonic plague by a
recombinant capsular
F1-V antigen
fusion
protein vaccine. Vaccine
1998;16:1131-7.
216.
Cecil RL, Austin JH.
Results of prophylactic
inoculation against
pneumococcus in 12,519
men. J Exp
Med
1918;28:19-41.
217.
Cecil RL, Vaughan HF.
Results of prophylactic
vaccination against
pneumonia at Camp
Wheeler. J Exp
Med
1919;29:457-83.
218.
MacLeod CM, Hodges RG,
Heidelberger M, Bernhard
WG. Prevention of
pneumococcal pneumonia
by
immunization with
specific capsular
polysaccharides. J Exp
Med 1945;81:445-65.
219.
English PC. Therapeutic
strategies to combat
pneumococcal disease:
Repeated failure of
physicians to
adopt
pneumococcal vaccine,
1900-1945. Perspect Biol
Med 1987; 30:170-85.
220.
McKeehan JA, Ryan MA,
Gray GC. Pneumococcal
vaccine to counter
emerging infectious
disease
threat
in the military. Mil Med
2001;166:1087-90.
221.
Advisory Committee on
Immunization Practices.
Poliomyelitis prevention
in the United States:
Updated
recommendations. MMWR
2000;49(RR-5):1-22.
ftp.cdc.gov/pub/Publications/mmwr/rr/rr4905.pdf
222.
Peeler RN, Cluff LE,
Trever RW.
Hyper-immunization of
man.
Bulletin of the Johns
Hopkins Hospital
1958;103:183-98.
www.vaccines.mil/documents/library/Peeler
1958 Bull J Hopk Hosp p.
183.pdf
223.
Haycraft RG. Studies on
hyperimmunization in
man. Mil Med
1962;127:745-52.
224.
Peeler RN, Kadull PJ,
Cluff LE. Intensive
immunization of man:
Evaluation of possible
adverse
consequences.
Annals of Internal
Medicine
1965;63:44-57.
www.anthrax.mil/media/pdf/Intensive.pdf
225.
White CS III, Adler WH,
McGann VG. Repeated
immunization: Possible
adverse effects:
Reevaluation
of human
subjects at 25 years.
Ann Intern Med
1974;81:594-600.
www.anthrax.mil/media/pdf/Repeated.pdf
226.
Rusnak JM, Kortepeter
MG, Aldis J, Boudreau E.
Experience in the
medical management of
potential
laboratory exposures to
agents of bioterrorism
on the basis of risk
assessment at the United
States Army
Medical
Research Institute of
Infectious Diseases (USAMRIID).
J Occup Environ Med
2004;46:801-11.
227.
Pittman PR, Norris SL,
Coonan KM, McKee KT Jr.
An assessment of health
status among medical
research
volunteers who served in
the Project Whitecoat
program at Fort Detrick,
Maryland. Mil Med
2005;170:183-7.
228. Sadusk JF Jr. Typhus fever in the United States Army following
immunization: Incidence, severity of thedisease, modification of
the clinical course and
serologic diagnosis.
JAMA 1947;133:1192-9.
229.
Bayne-Jones S.
Commentary on typhus
control in World War II.
Yale J Biol Med
1950;22:483-93.
230.
Bayne-Jones S. Typhus
fevers. In: Preventive
Medicine in World War
II, Communicable
Diseases, vol.
VII,
Communicable Diseases.
Washington, DC, Office
of the Surgeon General,
Department of the Army,
1964.
231.
Bavaro MF, Kelly DJ,
Dasch GA, Hale BR, Olson
P. History of U.S.
military contributions
to the study of
rickettsial diseases.
Mil Med
2005;170(Suppl):49-60.
232.
McNeil JG, Lednar WM,
Stansfield SK, Prier RE,
Miller RN. Central
European tick-borne
encephalitis:
Assessment of risk for
persons in the Armed
Forces and vacationers.
J Infect Dis
1985;152:650-1.
233.
Craig SC, Pittman PR,
Lewis TE, Rossi CA,
Henchal EA, Kuschner RA,
Martinez C, Kohlhase KF,
Cuthie
JC, Welch GE, Sanchez
JL. An accelerated
schedule for tick-borne
encephalitis vaccine:
The
American
Military experience in
Bosnia. Am J Trop Med
Hyg 1999;61:874-8.
234.
Bruton OC.
Agammaglobulinemia.
Pediatrics 1952;9:722-8.
235.
Bruton OC. A decade with
agammaglobulinemia. J
Pediatr 1962;60:672-6.
236.
Buckley CR.
Agammaglobulinemia, by
Col. Ogden C. Bruton,
MC, USA,
Pediatrics,
1952;9:722-728.
Pediatrics 1998;102(1 Pt
2):213-5.
237.
Kelso JM, Jones RT,
Yunginger JW.
Anaphylaxis to measles,
mumps, and rubella
vaccine mediated by
IgE to
gelatin. J Allergy Clin
Immunol 1993;91:867-72
238.
Agerty HA. An outbreak
of smallpox in American
military personnel in
Japan. N Engl J Med
1947;237:305-9.
239.
National Vaccine
Advisory Committee.
Adult immunization
programs in
nontraditional settings:
Quality
standards and guidance
for program evaluation.
MMWR 2000;49(RR-1):1-13.
ftp.cdc.gov/pub/Publications/mmwr/rr/rr4901.pdf
240.
Armed Forces
Epidemiological Board.
Recommendation 2004-04:
Multiple concurrent
immunizations
and
safety concerns. Falls
Church, VA: 16 April
2004.
www.vaccines.mil/documents/477AFEB2004.pdf
241.
Institute of Medicine.
Immunization Safety
Review: Multiple
Immunizations and Immune
Dysfunction.
Washington, DC: National
Academy of Sciences, Feb
2002.
www.nap.edu/catalog/10306.html
242.
Offit PA, Quarles J,
Gerber MA, Hackett CJ,
Marcuse EK, Kollman TR,
Gellin BG, Landry S.
Addressing parents'
concerns: Do multiple
vaccines overwhelm or
weaken the infant's
immune system?
Pediatrics
2002;109:124-9.
243.
Paul JR. A nationwide
serum survey of US
recruits in the three
Armed Services. Mil Med
1965;130:1-
12.
244.
Jacobs RJ, Saab S,
Meyerhoff AS, Koff RS.
An economic assessment
of pre-vaccination
screening for
hepatitis A and B.
Public Health Rep
2003;118:550-8.
245.
Scott PT, Niebuhr DW,
McGready JB, Gaydos JC.
Hepatitis B immunity in
United States military
recruits. J Infect Dis
2005;191:1835-41.
246.
Pablo K, Rooks P, Nevin
R. Benefits of serologic
screening for hepatitis
B immunity in military
recruits. J
Infect
Dis 2005;191:2180-1.
247.
Warren J, Ziherl FA,
Kish AW, Ziherl LA.
Large-scale
administration of
vaccines by means of an
automatic jet injection
syringe. JAMA
1953;157:633-7.
248.
Benenson AS. Mass
immunization by jet
injection. Proceedings
of the International
Symposium of
Immunology,
International Committee
for Microbiological
Standardization,
Opatija, Yugoslavia,
1959:393-
9.
249.
Hingson RA, Davis HS,
Rosen M. The historical
development of jet
injection and envisions
uses in
mass
immunization and mass
therapy based upon two
decades’ experience. Mil
Med 1963;128:516-24.
250.
Hingson RA, Davis HS,
Rosen. Clinical
experience with one and
a half million jet
injections in parenteral
therapy
and in preventive
medicine. Mil Med
1963;128:525-8.
251.
Foege WH, Eddins DL.
Mass vaccination
programs in developing
countries. Prog Med
Virol
1973;15:205-43.
252.
Centers for Disease
Control & Prevention.
Hepatitis B associated
with jet gun
injection—California.
MMWR
1986;35:272-6.
253.
Canter J, Mackey K, Good
LS, Roberto RR, Chin J,
Bond WW, Alter MJ, Horan
JM. An outbreak of
hepatitis B associated
with jet injections in a
weight reduction clinic.
Arch Intern Med
1990;150:1923-7.
254.
Borio L, Inglesby T,
Peters CJ, Schmaljohn
AL, Hughes JM, Jahrling
PB, Ksiazek T, Johnson
KM,
Meyerhoff A, O'Toole T,
Ascher MS, Bartlett J,
Breman JG, Eitzen EM Jr,
Hamburg M, Hauer J,
Henderson DA, Johnson
RJ, Kwik G, Layton M,
Lillibridge S, Nabel GJ.
Hemorrhagic fever
viruses as
Immunization to Protect
the U.S. Armed Forces
37
biological weapons:
Medical and public
health management. JAMA
2002;287:2391-405.
jama.amaassn.
org/cgi/reprint/287/18/2391.pdf
255.
Maiztegui JI, McKee KT
Jr, Barrera Oro JG,
Harrison LH, Gibbs PH,
Feuillade MR, Enria DA,
Briggiler
AM,
Levis SC, Ambrosio AM,
Halsey NA, Peters CJ.
Protective efficacy of a
live attenuated vaccine
against
Argentine hemorrhagic
fever. J Infect Dis
1998;177:277-83.
256.
Byrne MP, Smith LA.
Development of vaccines
for prevention of
botulism. Biochimie
2000;82:955-66.
257.
Levitt NH, Ramsburg HH,
Hasty SE, Repik PM, Cole
FE Jr, Lupton HW.
Development of an
attenuated
strain
of chikungunya virus for
use in vaccine
production. Vaccine
1986;4:157-62.
258.
Edelman R, Tacket CO,
Wasserman SS, Bodison
SA, Perry JG, Mangiafico
JA. Phase II safety and
immunogenicity study of
live chikungunya virus
vaccine TSI-GSD-218. Am
J Trop Med Hyg
2000;62:681-
5.
259.
Innis BL, Eckels KH.
Progress in development
of a live-attenuated,
tetravalent dengue virus
vaccine by
the
United States Army
Medical Research and
Materiel Command. Am J
Trop Med Hyg 2003;69(6
Suppl):1-4. Erratum
2004;70:336.
260.
Bartelloni PJ, McKinney
RW, Duffy TP, Cole FE
Jr. An inactivated
eastern equine
encephalomyelitis
vaccine
propagated in
chick-embryo cell
culture: II. Clinical
and serologic responses
in man. Am J Trop
Med Hyg
1970;19:123-6.
261.
Pushko P, Geisbert J,
Parker M, Jahrling P,
Smith J. Individual and
bivalent vaccines based
on
alphavirus replicons
protect guinea pigs
against infection with
Lassa and Ebola viruses.
J Virol
2001;75:11677-85.
262.
Jones SM, Feldmann H,
Stroher U, Geisbert JB,
Fernando L, Grolla A,
Klenk HD, Sullivan NJ,
Volchkov
VE,
Fritz EA, Daddario KM,
Hensley LE, Jahrling PB,
Geisbert TW. Live
attenuated recombinant
vaccine
protects
nonhuman primates
against Ebola and
Marburg viruses. Nat Med
2005;11:786-90.
263.
Katz DE, DeLorimier AJ,
Wolf MK, Hall ER,
Cassels FJ, van Hamont
JE, Newcomer RL, Davachi
MA,
Taylor
DN, McQueen CE. Oral
immunization of adult
volunteers with
microencapsulated
enterotoxigenic
Escherichia coli (ETEC)
CS6 antigen. Vaccine
2003;21:341-6.
264.
Emerson SU, Purcell RH.
Hepatitis E virus. Rev
Med Virol
2003;13:145-54.
265.
Birx DL, Loomis-Price
LD, Aronson N, Brundage
J, Davis C, Deyton L,
Garner R, Gordin F,
Henry D,
Holloway
W, Kerkering T,
Luskin-Hawk R, McNeil J,
Michael N, Foster Pierce
P, Poretz D, Ratto-Kim
S,
Renzullo
P, Ruiz N, Sitz K, Smith
G, Tacket C, Thompson M,
Tramont E, Yangco B,
Yarrish R, Redfield
RR.
Efficacy testing of
recombinant human
immunodeficiency virus
(HIV) gp160 as a
therapeutic vaccine
in
early-stage
HIV-1-infected
volunteers. J Infect Dis
2000;181:881-9.
266.
Nitayaphan S,
Khamboonruang C,
Sirisophana N, Morgan P,
Chiu J, Duliege AM,
Chuenchitra C,
Supapongse T,
Rungruengthanakit K,
deSouza M, Mascola JR,
Boggio K, Ratto-Kim S,
Markowitz LE,
Birx D,
Suriyanon V, McNeil JG,
Brown AE, Michael RA. A
phase I/II trial of HIV
SF2 gp120/MF59
vaccine
in seronegative Thais.
Vaccine 2000;18:1448-55.
267.
Brown AE, Nitayaphan S.
Foundations for a Phase
III human
immunodeficiency virus
vaccine trial: A
decade
of Thai-U.S. Army
collaborative research.
Mil Med 2004;169:588-93.
268.
Rasnake MS, Conger NG,
McAllister K, Holmes KK,
Tramont EC. History of
U.S. military
contributions
to the
study of sexually
transmitted diseases.
Mil Med 2005;170(4
Suppl):61-5.
269.
Hockmeyer WT, Ballou WR.
Sporozoite immunity and
vaccine development.
Prog Allergy. 1988;41:1-
14.
270.
Alonso PL, Sacarlal J,
Aponte JJ, Leach A,
Macete E, Milman J,
Mandomando I, Spiessens
B,
Guinovart C, Espasa M,
Bassat Q, Aide P,
Ofori-Anyinam O, Navia
MM, Corachan S, Ceuppens
M,
Dubois
MC, Demoitie MA,
Dubovsky F, Menendez C,
Tornieporth N, Ballou
WR, Thompson R, Cohen J.
Efficacy
of the RTS,S/AS02A
vaccine against
Plasmodium falciparum
infection and disease in
young
African
children: randomised
controlled trial. Lancet
2004;364:1411-20.
271.
Heppner DG Jr, Kester
KE, Ockenhouse CF,
Tornieporth N, Ofori O,
Lyon JA, Stewart VA,
Dubois P,
Lanar
DE, Krzych U, Moris P,
Angov E, Cummings JF,
Leach A, Hall BT, Dutta
S, Schwenk R, Hillier C,
Barbosa
A, Ware LA, Nair L,
Darko CA, Withers MR,
Ogutu B, Polhemus ME,
Fukuda M, Pichyangkul S,
Gettyacamin M, Diggs C,
Soisson L, Milman J,
Dubois MC, Garcon N,
Tucker K, Wittes J,
Plowe CV,
Thera
MA, Duombo OK, Pau MG,
Goudsmit J, Ballou WR,
Cohen J. Towards an
RTS,S-based, multistage,
multi-antigen vaccine
against falciparum
malaria: Progress at the
Walter Reed Army
Institute of
Research. Vaccine
2005;23:2243-50.
272.
Ockenhouse CF, Magill A,
Smith D, Milhous W.
History of U.S. military
contributions to the
study of
malaria.
Mil Med 2005;170(4
Suppl):12-6.
273.
Mogabgab WJ. Protective
effects of inactive
Mycoplasma pneumoniae
vaccine in military
personnel,
Immunization to Protect
the U.S. Armed Forces
38
1964-1966. Am Rev Resp
Dis 1968;97:359-65.
274.
Boslego J, Garcia J,
Cruz C, Zollinger W,
Brandt B, Ruiz S,
Martinez M, Arthur J,
Underwood P, Silva
W, Moran
W, Hankins W, Gilly J,
Mays J, Chilean National
Committee for
Meningococcal Disease.
Efficacy, safety, and
immunogenicity of a
meningococcal group B
(15:P1.3) outer membrane
protein
vaccine
in Iquique, Chile.
Vaccine 1995;13:821-9.
275.
Katial RK, Brandt BL,
Moran EE, Marks S,
Agnello V, Zollinger WD.
Immunogenicity and
safety testing
of a
group B intranasal
meningococcal native
outer membrane vesicle
vaccine. Infect Immun
2002;70:702-7.
276.
Vivona S, Lowenthal JP,
Berman S, Benenson AS,
Smadel JE. Report of a
field study with Q fever
vaccine.
Am J Hyg 1964;79:143-53.
277.
Waag DM, England MJ,
Tammariello RF, Byrne
WR, Gibbs P, Banfield
CM, Pitt ML. Comparative
efficacy
and immunogenicity of Q
fever
chloroform:methanol
residue (CMR) and phase
I cellular (Q-Vax)
vaccines
in cynomolgus monkeys
challenged by aerosol.
Vaccine 2002;20:2623-34.
278.
Randall R, Gibbs CJ Jr,
Aulisio CG, Binn LN,
Harrison VR. The
development of a
formalin-killed Rift
Valley
fever virus vaccine for
use in man.
J. Immunol
1962;89:660-71.
279.
Randall R, Binn LN,
Harrison VR.
Immunization against
Rift Valley fever virus,
studies on the
immunogenicity of
lyophilized
formalin-inactivated
vaccine.
J Immunol
1964;93:293-9.
280.
Niklasson B. Rift Valley
fever virus vaccine
trial: Study of
side-effects in humans.
Scand J Infect Dis
1982;14:105-9.
281.
Niklasson B, Peters CJ,
Bengtsson E, Norrby E.
Rift Valley fever virus
vaccine trial: Study of
neutralizing antibody
response in humans.
Vaccine 1985;3:123-7.
282.
Pittman PR, Liu CT,
Cannon TL, Makuch RS,
Mangiafico JA, Gibbs PH,
Peters CJ.
Immunogenicity of
an
inactivated Rift Valley
fever vaccine in humans:
A 12-year experience.
Vaccine 1999;18:181-9.
283.
Katz DE, Coster TS, Wolf
MK, Trespalacios FC,
Cohen D, Robins G,
Hartman AB, Venkatesan
MM,
Taylor
DN, Hale TL. Two studies
evaluating the safety
and immunogenicity of a
live, attenuated
Shigella
flexneri
2a vaccine (SC602) and
excretion of vaccine
organisms in North
American volunteers.
Infect
Immun
2004;72:923-30.
284.
Drabick JJ, Narayanan
RB, Williams JC, Leduc
JW, Nacy CA. Passive
protection of mice
against lethal
Francisella tularensis
(live tularemia vaccine
strain) infection by the
sera of human recipients
of the live
tularemia vaccine. Am J
Med Sci 1994;308:83-7.
285.
Waag DM, McKee KT Jr,
Sandstrom G, Pratt LL,
Bolt CR, England MJ,
Nelson GO, Williams JC.
Cellmediated
and
humoral immune responses
after vaccination of
human volunteers with
the live vaccine
strain
of Francisella
tularensis. Clin Diagn
Lab Immunol
1995;2:143-8.
286.
Waag DM, Sandstrom G,
England MJ, Williams JC.
Immunogenicity of a new
lot of Francisella
tularensis live vaccine
strain in human
volunteers. FEMS Immunol
Med Microbiol
1996;13:205-9.
287.
Dennis DT, Inglesby TV,
Henderson DA, Bartlett
JG, Ascher MS, Eitzen E,
Fine AD, Friedlander AM,
Hauer J,
Layton M, Lillibridge
SR, McDade JE, Osterholm
MT, O'Toole T, Parker G,
Perl TM, Russell
PK,
Tonat K, Working Group
on Civilian Biodefense.
Tularemia as a
biological weapon:
Medical and
public
health management. JAMA
2001;285:2763-73.
jama.ama-assn.org/cgi/reprint/285/21/2763.pdf
288.
Alevizatos AC, McKinney
RW, Feigin RD. Live,
attenuated Venezuelan
equine encephalomyelitis
virus
vaccine.
I. Clinical effects in
man. Am J Trop Med Hyg
1967;16:762-8.
289.
Pedersen CE Jr.
Preparation and testing
of vaccines prepared
from the envelopes of
Venezuelan,
eastern,
and western equine
encephalomyelitis
viruses. J Clin
Microbiol 1976;3:113-8.
290.
Pratt WD, Davis NL,
Johnston RE, Smith JF.
Genetically engineered,
live attenuated vaccines
for
Venezuelan equine
encephalitis: Testing in
animal models. Vaccine
2003;21:3854-62.
291.
Bartelloni PJ, McKinney
RW, Calia FM, Ramsburg,
Cole FE Jr. Inactivated
western equine
encephalomyelitis
vaccine propagated in
chick embryo cell
culture: Clinical and
serological evaluation
in
man. Am
J Trop Med Hyg
1971;20:146-9.
292.
Boslego JW, Tramont EC,
Chung RC, McChesney DG,
Ciak J, Sadoff JC,
Piziak MV, Brown JD,
Brinton
CC Jr,
Wood SW, Bryan JR.
Efficacy trial of a
parenteral gonococcal
pilus vaccine in men.
Vaccine
1991;9:154-62.
293.
Reed DS, Lind CM,
Lackemeyer MG, Sullivan
LJ, Pratt WD, Parker MD.
Genetically engineered,
live,
attenuated vaccines
protect nonhuman
primates against aerosol
challenge with a
virulent IE strain of
Venezuelan equine
encephalitis virus.
Vaccine 2005;23:3139-47.
294.
Klinman DM, Xie H,
Little SF, Currie D,
Ivins BE. CpG
oligonucleotides improve
the protective immune
response
induced by the anthrax
vaccination of rhesus
macaques. Vaccine
2004;22:2881-6.
295.
Klinman DM, Currie D,
Gursel I, Verthelyi D.
Use of CpG
oligodeoxynucleotides as
immune adjuvants.
Immunization to Protect
the U.S. Armed Forces
39
Immunol
Rev 2004;199:201-16.
296. Xie
H, Gursel I, Ivins BE,
Singh M, O'Hagan DT,
Ulmer JB, Klinman DM.
CpG
oligodeoxynucleotides
adsorbed
onto
polylactide-co-glycolide
microparticles improve
the immunogenicity and
protective activity
of the
licensed anthrax
vaccine. Infect Immun
2005;73:828-33.
297.
Boles JW, Pitt ML,
LeClaire RD, Gibbs PH,
Ulrich RG, Bavari S.
Correlation of body
temperature with
protection against
staphylococcal
enterotoxin B exposure
and use in determining
vaccine dose-schedule.
Vaccine
2003;21:2791-6.
298.
Schmaljohn CS, Hasty SE,
Dalrymple JM.
Preparation of candidate
vaccinia-vectored
vaccines for
haemorrhagic fever with
renal syndrome. Vaccine
1992;10:10-3.
299.
McClain DJ, Summers PL,
Harrison SA, Schmaljohn
AL, Schmaljohn CS.
Clinical evaluation of a
vaccinia-vectored
Hantaan virus vaccine. J
Med Virol 2000;60:77-85.
300.
Schoepp RJ, Smith JF,
Parker MD. Recombinant
chimeric western and
eastern equine
encephalitis
viruses
as potential vaccine
candidates. Virology
2002;302:299-309.
301.
Hevey M, Negley D,
Pushko P, Smith J,
Schmaljohn A. Marburg
virus vaccines based
upon alphavirus
replicons protect guinea
pigs and nonhuman
primates. Virology
1998;251:28-37.
302.
Hevey M, Negley D,
VanderZanden L,
Tammariello RF, Geisbert
J, Schmaljohn C, Smith
JF, Jahrling
PB,
Schmaljohn AL. Marburg
virus vaccines:
Comparing classical and
new approaches. Vaccine
2001;20:586-93.
303.
Custer DM, Thompson E,
Schmaljohn CS, Ksiazek
TG, Hooper JW. Active
and passive vaccination
against
hantavirus pulmonary
syndrome with Andes
virus M genome
segment-based DNA
vaccine. J
Virol
2003;77:9894-905.
304.
McClain JB, Edelman R,
Shmuklarsky M, Que J,
Cryz SJ, Cross AS.
Unusual persistence in
healthy
volunteers and ill
patients of hyperimmune
immunoglobulin directed
against multiple
Pseudomonas Ochain
and
Klebsiella serotypes
after intravenous
infusion. Vaccine
2001;19:3499-508.
305.
Maruyama T, Rodriguez
LL, Jahrling PB, Sanchez
A, Khan AS, Nichol ST,
Peters CJ, Parren PW,
Burton
DR. Ebola virus can be
effectively neutralized
by antibody produced in
natural human infection.
J
Virol
1999;73:6024-30.
306.
Eddy GA, Wagner FS,
Scott SK, Mahlandt BJ.
Protection of monkeys
against Machupo virus by
the
passive
administration of
Bolivian haemorrhagic
fever immunoglobulin
(human origin). Bull
World Health
Organ
1975;52:723-7.
307.
Hibbs RG, Weber JT,
Corwin A, Allos BM, Abd
el Rehim MS, Sharkawy
SE, Sarn JE, McKee KT
Jr.
Experience with the use
of an investigational
F(ab')2 heptavalent
botulism immune globulin
of equine
origin
during an outbreak of
type E botulism in
Egypt. Clin Infect Dis
1996;23:337-40.
308.
Hemming VG, Prince GA,
Rodriguez W, Kim HW,
Brandt CD, Parrott RH,
London WT, Ficher GW,
Baron
PA, Henson SA.
Respiratory syncytial
virus infections and
intravenous
gamma-globulins. Pediatr
Infect
Dis J 1988;7(5 Suppl):S103-6.
309.
Meegan JM. The Rift
Valley fever epizootic
in Egypt 1977-1978. I.
Description of the
epizootic and
virological studies.
Trans Roy Soc Trop
Med Hyg
1979;73:618-623.
310.
Centers for Disease
Control & Prevention.
Rift valley fever--East
Africa, 1997-1998. MMWR
1998;47:261-4.
311.
Kenyon RH, Sammons LS,
Pedersen CE Jr.
Comparison of three
Rocky Mountain spotted
fever
vaccines. J Clin
Microbiol 1975;2:300-4.
312.
Clements ML, Wisseman CL
Jr, Woodward TE, Fiset
P, Dumler JS, McNamee W,
Black RE, Rooney J,
Hughes
TP, Levine MM.
Reactogenicity,
immunogenicity, and
efficacy of a chick
embryo cell-derived
vaccine
for Rocky Mountain
spotted fever. J Infect
Dis 1983;148:922-30.
313.
Alving CR. Design and
selection of vaccine
adjuvants: Animal models
and human trials.
Vaccine
2002;20
(Suppl 3):S56-64.
|
|